Menu
Publications
2024
2023
2022
2021
2020
2019
2018
2017
2016
2015
2014
2013
2012
2011
2010
2009
2008
2007
2006
2005
2004
2003
2002
2001
Editor-in-Chief
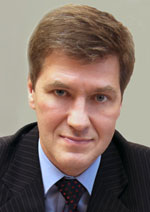
Nikiforov
Vladimir O.
D.Sc., Prof.
Partners
doi: 10.17586/2226-1494-2022-22-2-223-231
Dynamic range restrictions influence of the fiber-optic towed seismic streamer on the seismogram quality
Read the full article

Article in Russian
For citation:
Abstract
For citation:
Arzhanenkova A.N., Plotnikov M.Yu., Miroshnichenko G.P., Dmitraschenko P.Yu. Investigation of the dynamic range restrictions influence of the fiber-optic towed seismic streamer on the seismogram quality. Scientific and Technical Journal of Information Technologies, Mechanics and Optics, 2022, vol. 22, no. 2, pp. 223–231 (in Russian). doi: 10.17586/2226-1494-2022-22-2-223-231
Abstract
In this paper, we presented results of influence of the dynamic range in fiber-optic streamer signal processing circuit on the quality of recorded seismograms. By contrast with the existing hydroacoustic systems, which based on piezoceramic transducers, dynamic range limitations in a fiber-optic towed streamer do not lead to clipping of acoustic signals, but to complex nonlinear distortions that affect both the amplitude and phase frequency characteristics of the recorded signals. Therefore, main task of this work is to assess the distortion of seismograms obtained from acoustic signals recorded under limited dynamic range conditions of fiber-optic towed seismic streamer. To solve this problem, seismic signals obtained during field tests for various types of seismic streamers in the water area of Kola Bay are used. The recorded acoustic signals in the form of digital readings, converted into radians, taking into account the known sensitivity coefficient of hydrophones of the towed seismic streamer, are converted into digital reports of optical interference signals. Interference signals in digital form are equivalent to real optical signals recorded by the receiving path in the signal processing unit of the fiber optic streamer. The digital form of the recorded acoustic signals makes it possible to amplify them by multiplying them by a given gain factor, simulating various energy levels of an acoustic source. Further, these signals served as input data in the mathematical model of the signal processing circuit of the fiber-optic towed streamer, which takes into account the fundamental limitations of the dynamic range due to the finite sampling frequency of the interference signal, the fixed frequency of the auxiliary phase modulation, and the finite bandwidth of the low-pass filters used. Thus, it is possible to simulate the process of recording acoustic signals of a fiber-optic towed seismic streamer both without distortion and under conditions of limited dynamic range. As a result, signals from the output of the processing circuit model are used to construct seismograms of the same shelf area with different levels of acoustic amplification using the reflected wave method. The results demonstrate that the limitations of the dynamic range of signal processing circuit of fiber-optic towed seismic streamer have a significant impact on the quality of seismograms, on reducing the signals detailing, and also on decreasing the amplitudes of the recorded waves (in the presented data, the amplitude decreases by 5 times). The quality of seismograms drops significantly in areas of sharp transition between layers with different densities, which generate the most distinct and strong reflected seismic vibrations. The results obtained are of great practical importance, since they allow evaluating the effect of complex nonlinear distortions of acoustic signals under the conditions of limited dynamic range of the signal processing circuit of a fiber-optic towed seismic streamer on the received seismograms. These results are presented for the first time due to the lack of world analogues of the developed fiber-optic towed seismic streamer. In addition, taking into account the known sensitivity of the fiber-optic hydrophones of the streamer, the constructed model of the signal processing path allows choosing the optimal energy of the acoustic source for seismic exploration with the most efficient use of the dynamic range of the fiber-optic streamer.
Keywords: fiber-optic streamer, seismoacoustic research, interferometric measurements, dynamic range, reflected wave method, common depth point
Acknowledgements. This work was done in ITMO University and was financially supported by the Government of the Russian Federation (The Federal academic leadership program “Priority 2030”).
References
Acknowledgements. This work was done in ITMO University and was financially supported by the Government of the Russian Federation (The Federal academic leadership program “Priority 2030”).
References
-
Kirkendall C., Barock T., Tveten A., Dandridge A. Fiber optic towed arrays. Technical Report. Naval Research Laboratory, Washington, DC, USA, 2007.
-
Souto F. Fibre optic towed array: The high tech compact solution for naval warfare. Proc. of the Annual Conference of the Australian Acoustical Society 2013, Acoustics 2013: Science, Technology and Amenity, 2013, pp. 297–301.
-
Stolkin R., Sutin A., Radhakrishnan S., Bruno M., Fullerton B., Ekimov A., Raftery M. Feature based passive acoustic detection of underwater threats. Proceedings of SPIE, 2006, vol. 6204, pp. 620408. https://doi.org/10.1117/12.663651
-
Crickmore R.I., Nash P.J., Wooler J.P.F. Fiber optic security systems for land- and sea-based applications. Proceedings of SPIE, 2004, vol. 5611, pp. 79–87. https://doi.org/10.1117/12.578904
-
Borowski B., Sutin A., Roh H.-S., Bunin B. Passive acoustic threat detection in estuarine environments. Proceedings of SPIE, 2008, vol. 6945, pp. 694513. https://doi.org/10.1117/12.779177
-
De Freitas J.M. Recent developments in seismic seabed oil reservoir monitoring applications using fibre-optic sensing networks. Measurement Science and Technology, 2011, vol. 22, no. 5, pp. 052001. https://doi.org/10.1088/0957-0233/22/5/052001
-
Zhang M., Ma X., Wang L., Lai S., Zhou H., Zhao H., Liao Y. Progress of optical fiber sensors and its application in harsh environment. Photonic Sensors, 2011, vol. 1, no. 1, pp. 84–89. https://doi.org/10.1007/s13320-010-0012-1
-
Paulsson B.N.P., Toko J.L., Thornburg J.A., Slopko F., He R., Zhang C. A high performance fiber optic seismic sensor system. Proc. of the 38th Workshop Geothermal Reservoir Engineering, 2013, pp. 8.
-
Kringlebotn J.T., Nakstad H., Morten E. Fibre optic ocean bottom seismic cable system: From innovation to commercial success. Proceedings of SPIE, 2009, vol. 7503, pp. 75037U.
-
Berg C., Langhammer J., Nash P. Lifetime stability and reliability of fibre-optic seismic sensors for permanent reservoir monitoring. Proc. of the Society of Exploration Geophysicists International Exposition and 82nd Annual Meeting (SEG), 2012, P. 1472–1476. https://doi.org/10.1190/segam2012-1236.1
-
Meng Z., Chen W., Wang J., Hu X., Chen M., Zhang Y. Recent progress in fiber-optic hydrophones. Photonic Sensors, 2021, vol. 11, no. 1, pp. 109–122. https://doi.org/10.1007/s13320-021-0618-5
-
Fiber Optic Sensors: An Introduction for Engineers and Scientists. Ed. by E. Udd, W.B. Spillman. Hoboken, NJ, USA: John Wiley & Sons, 2011, 512 p.
-
Yin S., Ruffin P.B., Yu F.T.S. Fiber Optic Sensors. 2nd ed. Boca Raton, FL, USA: CRC Press, 2008, 496 p.
-
Dmitrashchenko P.Yu., Plotnikov M.Yu., Lavrov V.S., Volkov A.V., Sharkov I.A., Godovova A.S. Compensation of the towing noise of a fiber-optic streamer using an additional interferometer. Journal of Optical Technology, 2021, vol. 88, no. 9, pp. 532–535. https://doi.org/10.1364/JOT.88.000532
-
Cranch G.A., Nash P.J. Large-scale multiplexing of interferometric fiber-optic sensors using TDM and DWDM. Journal of Lightwave Technology, 2001, vol. 19, no. 5, pp. 687–699. https://doi.org/10.1109/50.923482
-
Kersey D., Dandridge A. Comparative analysis of multiplexing techniques for interferometric fiber sensors. Proceedings of SPIE, 1989, vol. 1120, pp. 236–246. https://doi.org/10.1117/12.960993
-
Belikin M.N., Plotnikov M.Yu., Strigalev V.E., Kulikov A.V., Kireenkov A.Yu. Experimental comparison of homodyne demodulation algorithms for phase fiber-optic sensor. Scientific and Technical Journal of Information Technologies, Mechanics and Optics, 2015, vol. 15, no. 6, pp. 1008–1014. (in Russian). https://doi.org/10.17586/2226-1494-2015-15-6-1008-1014
-
Christian T.R., Frank P.A., Houston B.H. Real-time analog and digital demodulator for interferometric fiber optic sensors. Proceedings of SPIE, 1994, vol. 2191, pp. 324–336. https://doi.org/10.1117/12.173962
-
Dandridge A., Tveten A.B., Giallorenzi T.G. Homodyne demodulation scheme for fiber optic sensors using phase generated carrier. IEEE Journal of Quantum Electronics, 1982, vol. 18, no. 10, pp. 1647–1653. https://doi.org/10.1109/JQE.1982.1071416
-
He J., Wang L., Li F., Liu Y. An ameliorated phase generated carrier demodulation algorithm with low harmonic distortion and high stability. Journal of Lightwave Technology, 2010, vol. 28, no. 22, pp. 3258–3265. https://doi.org/10.1109/JLT.2010.2081347
-
Tong Y., Zeng H., Li L., Zhou Y. Improved phase generated carrier demodulation algorithm for eliminating light intensity disturbance and phase modulation amplitude variation. Applied Optics, 2012, vol. 51, no. 29, pp. 6962–6967. https://doi.org/10.1364/AO.51.006962
-
Yan L., Zhang Y., Xie J., Lou Y., Chen B., Zhang S., Zhou Y. Nonlinear error compensation of PGC demodulation with the calculation of carrier phase delay and phase modulation depth. Journal of Lightwave Technology, 2021, vol. 39, no. 8, pp. 2327–2335. https://doi.org/10.1109/JLT.2021.3049481
-
Sun Z., Liu K., Jiang J., Xu T., Wang S., Guo H., Zhou Z., Xue K., Huang Y., Liu T. Dynamic phase extraction in an ameliorated distributed vibration sensor using a highly stable homodyne detection. IEEE Sensors Journal, 2021, vol. 21, no. 23, pp. 27005–27014. https://doi.org/10.1109/JSEN.2021.3122133
-
Plotnikov M.J., Kulikov A.V., Strigalev V.E., Meshkovsky I.K. Dynamic range analysis of the phase generated carrier demodulation technique. Advances in Optical Technologies, 2014, pp. 815108. https://doi.org/10.1155/2014/815108
-
Plotnikov M.Y., Lavrov V.S., Dmitraschenko P.Y., Kulikov A.V., Meshkovskiy I.K. Thin cable fiber-optic hydrophone array for passive acoustic surveillance applications. IEEE Sensors Journal, 2019, vol. 19, no. 9, pp. 3376–3382. https://doi.org/10.1109/JSEN.2019.2894323
-
Rissons A., Mollier J.C. The vertical-cavity surface emitting laser (VCSEL) and electrical access contribution. Optoelectronics-Devices and Applications, 2011, pp. 227–254. https://doi.org/10.5772/18299
-
Gdeisat M., Lilley F. One-Dimensional Phase Unwrapping Problem. Liverpool John Moores University, 2012.
-
Volkov A.V., Plotnikov M.Y., Mekhrengin M.V., Miroshnichenko G.P., Aleynik A.S. Phase modulation depth evaluation and correction technique for the PGC demodulation scheme in fiber-optic interferometric sensors. IEEE Sensors Journal, 2017, vol. 17, no. 13, pp. 4143–4150. https://doi.org/10.1109/JSEN.2017.2704287
-
Wang G.Q., Xu T.W., Li F. PGC demodulation technique with high stability and low harmonic distortion. IEEE Photonics Technology Letters, 2012, vol. 24, no. 23, pp. 2093–2096. https://doi.org/10.1109/LPT.2012.2220129
-
Hou L., Peng F., Yang J., Yuan Y., Yan D., Wu B., Yuan L. An improved PGC demodulation method to extend dynamic range and compensate low-frequency drift of modulation depth. Proceedings of SPIE, 2015, vol. 9634, pp. 96345Y. https://doi.org/10.1117/12.2195867
-
Boganik G.N., Gurvich I.I. Seismic Surveying. Tver, AIS Publ., 2006, 744 с. (in Russian)
-
Gainanov V.G. Workshop on Seismic Data Processing Using Reflection Wave Method and CMP-method. A Guide to Practical Exercises for the Course "Seismic Surveying". 2nd ed., Moscow, KDU, Dobrosvet Publ., 2018.(in Russian)