Menu
Publications
2024
2023
2022
2021
2020
2019
2018
2017
2016
2015
2014
2013
2012
2011
2010
2009
2008
2007
2006
2005
2004
2003
2002
2001
Editor-in-Chief
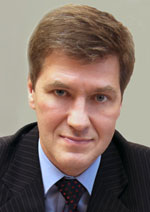
Nikiforov
Vladimir O.
D.Sc., Prof.
Partners
doi: 10.17586/2226-1494-2023-23-5-911-919
Switching the electrical properties of thin-film memristive elements based on GeTe by sequences of ultrashort laser pulses
Read the full article

Article in Russian
For citation:
Abstract
For citation:
Eliseev N.N., Nevzorov A.A., Mikhalevsky V.A., Kiselev A.V., Burtsev A.A., Ionin V.V., Lotin A.A. Switching the electrical properties of thin-film memristive elements based on GeTe by sequences of ultrashort laser pulses. Scientific and Technical Journal of Information Technologies, Mechanics and Optics, 2023, vol. 23, no. 5, pp. 911–919 (in Russian). doi: 10.17586/2226-1494-2023-23-5-911-919
Abstract
The work is devoted to the study of the characteristics of the state control of a thin-film element based on a phase-change GeTe material. The properties of such an element have been controlled by the action of sequences of ultrashort laser pulses. This action leads to a rapid heating of the thin film element and provides a phase transition between states with a resistance different by several orders of magnitude. The dynamics of the resistance was studied using a high speed oscilloscope according to the scheme where the element under study was the voltage divider arm of a highly stable source. Three different types of conductivity switching were observed for 100 nm thin films. For low energy laser radiation, several distinct states were obtained in which the material film has predominantly semiconducting properties. As the energy of the optical pulses increases, the number of possible stable states determined by the specific conductivity of the material decreases to two, one of which (low resistance) is exclusively metallic properties. In all cases, the time taken to switch to a stable state does not exceed a few tens of nanoseconds for films up to 100 nm thick. The study has demonstrated that the structures described can be used to implement optically controlled memristive elements. In addition, the large number of possible allowable specific resistances of the element will make it possible to use it to increase the information capacity of memory cells based on phase-change materials or to implement optoelectronic neuromorphic systems.
Keywords: phase-change materials, germanium telluride, thin films, neuromorphic elements, phase-change memory, memristors, optoelectronic memristors
Acknowledgements. This work was performed within the State assignment of the Federal Scientific Research Center “Crystallography and Photonics” of the Russian Academy of Sciences.
References
Acknowledgements. This work was performed within the State assignment of the Federal Scientific Research Center “Crystallography and Photonics” of the Russian Academy of Sciences.
References
- Le Gallo M., Sebastian A. An overview of phase-change memory device physics. Journal of Physics D: Applied Physics, 2020, vol. 53, no. 21, pp. 213002. https://doi.org/10.1088/1361-6463/ab7794
- Wright C.D., Hosseini P., Diosdado J.A.V. Beyond von-neumann computing with nanoscale phase-change memory devices. Advanced Functional Materials, 2013, vol. 23, no. 18, pp. 2248–2254. https://doi.org/10.1002/adfm.201202383
- Burr G.W., Kurdi B.N., Scott J.C., Lam C.H., Gopalakrishnan K., Shenoy R.S. Overview of candidate device technologies for storage-class memory. IBM Journal of Research and Development, 2008, vol. 52, no. 4.5, pp. 449–464. https://doi.org/10.1147/rd.524.0449
- Liu B., Wei T., Hu J., Li W., Ling Y., Liu Q., Cheng M., Song Z. Universal memory based on phase-change materials: From phase-change random access memory to optoelectronic hybrid storage. Chinese Physics B, 2021, vol. 30, no. 5, pp. 058504. https://doi.org/10.1088/1674-1056/abeedf
- Wuttig M., Yamada N. Phase-change materials for rewriteable data storage. Nature Materials, 2007, vol. 6, no. 11, pp. 824–832. https://doi.org/10.1038/nmat2009
- Song Z., Song S., Zhu M., Wu L., Ren K., Song W., Feng S. From octahedral structure motif to sub-nanosecond phase transitions in phase change materials for data storage. Science China Information Sciences, 2018, vol. 61, no. 8, pp. 081302. https://doi.org/10.1007/s11432-018-9404-2
- Pozidis H., Papandreou N., Sebastian A., Mittelholzer T., BrightSky M., Lam C., Eleftheriou E. A framework for reliability assessment in multilevel phase-change memory. Proc. of the 4th IEEE International Memory Workshop, 2012, pp. 1–4. https://doi.org/10.1109/IMW.2012.6213671
- Panin G.N. Optoelectronic dynamic memristor systems based on two-dimensional crystals. Chaos, Solitons & Fractals, 2021, vol. 142, pp. 110523. https://doi.org/10.1016/j.chaos.2020.110523
- Emboras A., Alabastri A., Lehmann P., Portner K., Weilenmann Ch., Ma P., Cheng B., Lewerenz M., Passerini E., Koch U., Aeschlimann J., Ducry F., Leuthold J., Luisier M. Opto-electronic memristors: Prospects and challenges in neuromorphic computing. Applied Physics Letters, 2020, vol. 117, no. 23, pp. 230502. https://doi.org/10.1063/5.0028539
- Photo-Electroactive Nonvolatile Memories for Data Storage and Neuromorphic Computing. Ed. by S. Han, Y. Zhou. Woodhead Publishing, 2020. https://doi.org/10.1016/C2019-0-00530-4
- Advances in Neuromorphic Memristor Science and Applications. Ed. by R. Kozma, R.E. Pino, G.E. Pazienza. Springer Science & Business Media Dordrecht, 2012. https://doi.org/10.1007/978-94-007-4491-2
- Shastri B.J., Tait A.N., Ferreira de Lima T., Pernice W.H.P., Bhaskaran H., Wright C.D., Prucnal P.R. Photonics for artificial intelligence and neuromorphic computing. Nature Photonics, 2021, vol. 15, no. 2, pp. 102–114. https://doi.org/10.1038/s41566-020-00754-y
- Bruns G., Merkelbach P., Schlockermann C., Salinga M., Wuttig M., Happ T.D., Philipp J.B., Kund M. Nanosecond switching in GeTe phase change memory cells. Applied Physics Letters, 2009, vol. 95, no. 4, pp. 043108. https://doi.org/10.1063/1.3191670
- Onodera A., Sakamoto I., Fujii Y., Mo⁁ri N., Sugai S. Structural and electrical properties of GeSe and GeTe at high pressure. Physical Review B, 1997, vol. 56, no. 13, pp. 7935–7941. https://doi.org/10.1103/PhysRevB.56.7935
- Fantini A., Perniola L., Armand M., Nodin J.F., Sousa V., Persico A., Cluzel J., Jahan C., Maitrejean S., Lhostis S., Roule A., Dressler C., Reimbold G., De Salvo B., Mazoyer P., Bensahel D., Boulanger F. Comparative assessment of GST and GeTe materials for application to embedded phase-change memory devices. Proc. of the 2009 IEEE International Memory Workshop, 2009, pp. 1–2. https://doi.org/10.1109/IMW.2009.5090585
- Singh K., Kumari S., Singh H., Bala N., Singh P., Kumar A., Thakur A. A review on GeTe thin film-based phase-change materials. Applied Nanoscience, 2023, vol. 13, no. 1, pp. 95–110. https://doi.org/10.1007/s13204-021-01911-7
- Perniola L., Sousa V., Fantini A., Arbaoui E., Bastard A., Armand M., Fargeix A., Jahan C., Nodin J.-F., Persico A., Blachier D., Toffoli A., Loubriat S., Gourvest E., Beneventi G.B., Feldis H., Maitrejean S., Lhostis S., Roule A., Cueto O., Reimbold G., Poupinet L., Billon T., De Salvo B., Bensahel D., Mazoyer P., Annunziata R., Zuliani P., Boulanger F. Electrical behavior of phase-change memory cells based on GeTe. IEEE Electron Device Letters, 2010, vol. 31, no. 5, pp. 488–490. https://doi.org/10.1109/LED.2010.2044136
- Eliseev N.N., Kiselev A.V., Ionin V.V., Mikhalevsky V.A., Burtsev A.A., Pankov M.A., Karimov D.N., Lotin A.A. Wide range optical and electrical contrast modulation by laser-induced phase transitions in GeTe thin films. Results in Physics, 2020, vol. 19, pp. 103466. https://doi.org/10.1016/j.rinp.2020.103466
- Gallard M., Amara M.S., Putero M., Burle N., Guichet Ch., Escoubas S., Richard M.-I., Mocuta C., Chahine R.R., Bernard M., Kowalczyk P., Noé P., Thomas O. New insights into thermomechanical behavior of GeTe thin films during crystallization. Acta Materialia, 2020, vol. 191, pp. 60–69. https://doi.org/10.1016/j.actamat.2020.04.001
- Berthier R., Cooper D., Sabbione C., Hippert F., Noé P. In situ observation of the impact of surface oxidation on the crystallization mechanism of GeTe phase-change thin films by scanning transmission electron microscopy. Journal of Applied Physics, 2017, vol. 122, no. 11, pp. 115304. https://doi.org/10.1063/1.5002637
- Coombs J.H., Jongenelis A.P.J.M., van Es-Spiekman W., Jacobs B.A.J. Laser‐induced crystallization phenomena in GeTe‐based alloys. I. Characterization of nucleation and growth.Journal of Applied Physics, 1995, vol. 78, no. 8, pp. 4906–4917. https://doi.org/10.1063/1.359779
- Wang W.J., Shi L.P., Zhao R., Lim K.G., Lee H.K., Chong T.C., Wu Y.H. Fast phase transitions induced by picosecond electrical pulses on phase change memory cells. Applied Physics Letters, 2008, vol. 93, no. 4, pp. 043121. https://doi.org/10.1063/1.2963196
- Edwards A.H., Pineda A.C., Schultz P.A., Martin M.G., Thompson A.P., Hjalmarson H.P. Theory of persistent, p-type, metallic conduction in c-GeTe. Journal of Physics: Condensed Matter, 2005, vol. 17, no. 32, pp. L329–L335. https://doi.org/10.1088/0953-8984/17/32/L01
- Wuttig M., Bhaskaran H., Taubner T. Phase-change materials for non-volatile photonic applications. Nature Photonics, 2017, vol. 11, no. 8, pp. 465–476. https://doi.org/10.1038/nphoton.2017.126
- Nevzorov A.A., Mikhalevsky V.A., Eliseev N.N., Kiselev A.V., Burtsev A.A., Ionin V.V., Maliutin A.M., Khmelenin D.N., Glebov V.N., Lotin A.A. Two-stage conductivity switching of GST thin films induced by femtosecond laser radiation. Optics & Laser Technology, 2023, vol. 157, pp. 108773. https://doi.org/10.1016/j.optlastec.2022.108773
- Ovshinsky S.R. Optical cognitive information processing – a new field. Japanese Journal of Applied Physics, 2004, vol. 43, no. 7S, pp. 4695. https://doi.org/10.1143/JJAP.43.4695
- Huber E., Marinero E.E. Laser-induced crystallization of amorphous GeTe: A time-resolved study. Physical Review B, 1987, vol. 36, no. 3, pp. 1595–1604. https://doi.org/10.1103/PhysRevB.36.1595
- Kiselev A.V., Mikhalevsky V.A., Burtsev A.A., Ionin V.V., Eliseev N.N., Lotin A.A. Transmissivity to reflectivity change delay phenomenon observed in GeTe thin films at laser-induced reamorphization. Optics & Laser Technology, 2021, vol. 143, pp. 107305. https://doi.org/10.1016/j.optlastec.2021.107305
- Hase M., Mizoguchi K., Nakashima S. Generation of coherent THz phonons in GeTe ferroelectrics. Journal of Luminescence, 2000, vol. 87-89, pp. 836–839. https://doi.org/10.1016/S0022-2313(99)00433-0
- Nath P., Chopra K.L. Thermal conductivity of amorphous and crystalline Ge and GeTe films. Physical Review B, 1974, vol. 10, no. 8, pp. 3412–3418. https://doi.org/10.1103/PhysRevB.10.3412
- Andrikopoulos K.S., Yannopoulos S.N., Voyiatzis G.A., Kolobov A.V., Ribes M., Tominaga J. Raman scattering study of the a-GeTe structure and possible mechanism for the amorphous to crystal transition. Journal of Physics: Condensed Matter, 2006, vol. 18, no. 3, pp. 965–979. https://doi.org/10.1088/0953-8984/18/3/014
- Burtsev A.A., Ionin V.V., Kiselev A.V., Eliseev N.N., Mikhalevsky V.A., Lotin A.A. Laser-induced crystallization kinetics of GeTe and Ge2Sb2Te5 thin films. Advanced Laser Technologies: Book of abstracts the 28th International Conference, 2021, pp. 81. https://doi.org/10.24412/cl-35039-2021-21-81-81
- Lu Q.M., Libera M. Microstructural measurements of amorphous GeTe crystallization by hot‐stage optical microscopy. Journal of Applied Physics, 1995, vol. 77, no. 2, pp. 517–521. https://doi.org/10.1063/1.359034
- Orava J., Greer A.L. Classical-nucleation-theory analysis of priming in chalcogenide phase-change memory. Acta Materialia, 2017, vol. 139, pp. 226–235. https://doi.org/10.1016/j.actamat.2017.08.013