Меню
Публикации
2025
2024
2023
2022
2021
2020
2019
2018
2017
2016
2015
2014
2013
2012
2011
2010
2009
2008
2007
2006
2005
2004
2003
2002
2001
Главный редактор
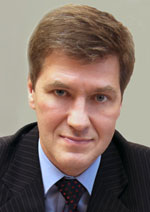
НИКИФОРОВ
Владимир Олегович
д.т.н., профессор
Партнеры
doi: 10.17586/2226-1494-2023-23-5-911-919
УДК 53.096
Переключение электрических свойств тонкопленочных мемристивных элементов на основе GeTe последовательностями сверхкоротких лазерных импульсов
Читать статью полностью

Язык статьи - русский
Ссылка для цитирования:
Аннотация
Ссылка для цитирования:
Елисеев Н.Н., Невзоров А.А., Михалевский В.А., Киселев А.В., Бурцев А.А., Ионин В.В., Лотин А.А. Переключение электрических свойств тонкопленочных мемристивных элементов на основе GeTe последовательностями сверхкоротких лазерных импульсов // Научно-технический вестник информационных технологий, механики и оптики. 2023. Т. 23, № 5. С. 911–919. doi: 10.17586/2226-1494-2023-23-5-911-919
Аннотация
Введение. Представлены результаты исследований особенностей управления состоянием тонкопленочного элемента на основе фазоизменяемого материала теллурида германия (GeTe). Управление свойствами элемента GeTe осуществлено последовательностями сверхкоротких лазерных импульсов. Такое воздействие приводит к быстрому нагреву тонкопленочного элемента и обеспечивает фазовый переход между состояниями с сопротивлением, отличным на несколько порядков. Метод. Динамика сопротивления исследована с помощью высокоскоростного осциллографа по схеме, где исследуемый элемент являлся плечом делителя напряжения высокостабильного источника. Основные результаты. Для пленок толщиной 100 нм определены три типа переключения удельной проводимости. Для низких энергий лазерного излучения получено несколько различимых состояний, при которых пленка материала обладает преимущественно полупроводниковыми свойствами. При увеличении энергии оптических импульсов количество возможных стабильных состояний, определяемых удельной проводимостью материала, уменьшена до двух, в одном из которых (низкоомном) материал проявил исключительно металлические свойства. Во всех случаях время переключения в стабильное состояние не превышает нескольких десятков наносекунд для пленок толщиной до 100 нм. Обсуждение. Исследование показало, что описанные структуры можно использовать для реализации мемристивных элементов с оптическим управлением. Кроме того, большое количество возможных допустимых удельных сопротивлений элемента позволит использовать его для увеличения информационной емкости ячеек памяти на основе фазоизменяемого материала или для реализации оптоэлектронных нейроморфных систем.
Ключевые слова: фазоизменяемые материалы, теллурид германия, тонкие пленки, нейроморфные элементы, память с изменением фазового состояния, мемристор, оптоэлектронные мемристоры
Благодарности. Работа выполнена в рамках Государственного задания Федерального научно-исследовательского центра «Кристаллография и фотоника» РАН.
Список литературы
Благодарности. Работа выполнена в рамках Государственного задания Федерального научно-исследовательского центра «Кристаллография и фотоника» РАН.
Список литературы
- Le Gallo M., Sebastian A. An overview of phase-change memory device physics // Journal of Physics D: Applied Physics. 2020. V. 53. N 21. P. 213002. https://doi.org/10.1088/1361-6463/ab7794
- Wright C.D., Hosseini P., Diosdado J.A.V. Beyond von-neumann computing with nanoscale phase-change memory devices // Advanced Functional Materials. 2013. V. 23. N 18. P. 2248–2254. https://doi.org/10.1002/adfm.201202383
- Burr G.W., Kurdi B.N., Scott J.C., Lam C.H., Gopalakrishnan K., Shenoy R.S. Overview of candidate device technologies for storage-class memory // IBM Journal of Research and Development. 2008. V. 52. N 4.5. P. 449–464. https://doi.org/10.1147/rd.524.0449
- Liu B., Wei T., Hu J., Li W., Ling Y., Liu Q., Cheng M., Song Z. Universal memory based on phase-change materials: From phase-change random access memory to optoelectronic hybrid storage // Chinese Physics B. 2021. V. 30. N 5. P. 058504. https://doi.org/10.1088/1674-1056/abeedf
- Wuttig M., Yamada N. Phase-change materials for rewriteable data storage // Nature Materials. 2007. V. 6. N 11. P. 824–832. https://doi.org/10.1038/nmat2009
- Song Z., Song S., Zhu M., Wu L., Ren K., Song W., Feng S. From octahedral structure motif to sub-nanosecond phase transitions in phase change materials for data storage // Science China Information Sciences. 2018. V. 61. N 8. P. 081302. https://doi.org/10.1007/s11432-018-9404-2
- Pozidis H., Papandreou N., Sebastian A., Mittelholzer T., BrightSky M., Lam C., Eleftheriou E. A framework for reliability assessment in multilevel phase-change memory // Proc. of the 4th IEEE International Memory Workshop. 2012. P. 1–4. https://doi.org/10.1109/IMW.2012.6213671
- Panin G.N. Optoelectronic dynamic memristor systems based on two-dimensional crystals // Chaos, Solitons & Fractals. 2021. V. 142. P. 110523. https://doi.org/10.1016/j.chaos.2020.110523
- Emboras A., Alabastri A., Lehmann P., Portner K., Weilenmann Ch., Ma P., Cheng B., Lewerenz M., Passerini E., Koch U., Aeschlimann J., Ducry F., Leuthold J., Luisier M. Opto-electronic memristors: Prospects and challenges in neuromorphic computing // Applied Physics Letters. 2020. V. 117. N 23. P. 230502. https://doi.org/10.1063/5.0028539
- Photo-Electroactive Nonvolatile Memories for Data Storage and Neuromorphic Computing / ed. by S. Han, Y. Zhou. Woodhead Publishing, 2020. https://doi.org/10.1016/C2019-0-00530-4
- Advances in Neuromorphic Memristor Science and Applications / ed. by R. Kozma, R.E. Pino, G.E. Pazienza. Springer Science & Business Media Dordrecht, 2012. https://doi.org/10.1007/978-94-007-4491-2
- Shastri B.J., Tait A.N., Ferreira de Lima T., Pernice W.H.P., Bhaskaran H., Wright C.D., Prucnal P.R. Photonics for artificial intelligence and neuromorphic computing // Nature Photonics. 2021. V. 15. N 2. P. 102–114. https://doi.org/10.1038/s41566-020-00754-y
- Bruns G., Merkelbach P., Schlockermann C., Salinga M., Wuttig M., Happ T.D., Philipp J.B., Kund M. Nanosecond switching in GeTe phase change memory cells // Applied Physics Letters. 2009. V. 95. N 4. P. 043108. https://doi.org/10.1063/1.3191670
- Onodera A., Sakamoto I., Fujii Y., Mo⁁ri N., Sugai S. Structural and electrical properties of GeSe and GeTe at high pressure // Physical Review B. 1997. V. 56. N 13. P. 7935–7941. https://doi.org/10.1103/PhysRevB.56.7935
- Fantini A., Perniola L., Armand M., Nodin J.F., Sousa V., Persico A., Cluzel J., Jahan C., Maitrejean S., Lhostis S., Roule A., Dressler C., Reimbold G., De Salvo B., Mazoyer P., Bensahel D., Boulanger F. Comparative assessment of GST and GeTe materials for application to embedded phase-change memory devices // Proc. of the 2009 IEEE International Memory Workshop. 2009. Р. 1–2. https://doi.org/10.1109/IMW.2009.5090585
- Singh K., Kumari S., Singh H., Bala N., Singh P., Kumar A., Thakur A. A review on GeTe thin film-based phase-change materials // Applied Nanoscience. 2023. V. 13. N 1. P. 95–110. https://doi.org/10.1007/s13204-021-01911-7
- Perniola L., Sousa V., Fantini A., Arbaoui E., Bastard A., Armand M., Fargeix A., Jahan C., Nodin J.-F., Persico A., Blachier D., Toffoli A., Loubriat S., Gourvest E., Beneventi G.B., Feldis H., Maitrejean S., Lhostis S., Roule A., Cueto O., Reimbold G., Poupinet L., Billon T., De Salvo B., Bensahel D., Mazoyer P., Annunziata R., Zuliani P., Boulanger F. Electrical behavior of phase-change memory cells based on GeTe // IEEE Electron Device Letters. 2010. V. 31. N 5. P. 488–490. https://doi.org/10.1109/LED.2010.2044136
- Eliseev N.N., Kiselev A.V., Ionin V.V., Mikhalevsky V.A., Burtsev A.A., Pankov M.A., Karimov D.N., Lotin A.A. Wide range optical and electrical contrast modulation by laser-induced phase transitions in GeTe thin films // Results in Physics. 2020. V. 19. P. 103466. https://doi.org/10.1016/j.rinp.2020.103466
- Gallard M., Amara M.S., Putero M., Burle N., Guichet Ch., Escoubas S., Richard M.-I., Mocuta C., Chahine R.R., Bernard M., Kowalczyk P., Noé P., Thomas O. New insights into thermomechanical behavior of GeTe thin films during crystallization // Acta Materialia. 2020. V. 191. P. 60–69. https://doi.org/10.1016/j.actamat.2020.04.001
- Berthier R., Cooper D., Sabbione C., Hippert F., Noé P. In situ observation of the impact of surface oxidation on the crystallization mechanism of GeTe phase-change thin films by scanning transmission electron microscopy // Journal of Applied Physics. 2017. V. 122. N 11. P. 115304. https://doi.org/10.1063/1.5002637
- Coombs J.H., Jongenelis A.P.J.M., van Es-Spiekman W., Jacobs B.A.J. Laser‐induced crystallization phenomena in GeTe‐based alloys. I. Characterization of nucleation and growth // Journal of Applied Physics. 1995. V. 78. N 8. P. 4906–4917. https://doi.org/10.1063/1.359779
- Wang W.J., Shi L.P., Zhao R., Lim K.G., Lee H.K., Chong T.C., Wu Y.H. Fast phase transitions induced by picosecond electrical pulses on phase change memory cells // Applied Physics Letters. 2008. V. 93. N 4. P. 043121. https://doi.org/10.1063/1.2963196
- Edwards A.H., Pineda A.C., Schultz P.A., Martin M.G., Thompson A.P., Hjalmarson H.P. Theory of persistent, p-type, metallic conduction in c-GeTe // Journal of Physics: Condensed Matter. 2005. V. 17. N 32. P. L329–L335. https://doi.org/10.1088/0953-8984/17/32/L01
- Wuttig M., Bhaskaran H., Taubner T. Phase-change materials for non-volatile photonic applications // Nature Photonics. 2017. V. 11. N 8. P. 465–476. https://doi.org/10.1038/nphoton.2017.126
- Nevzorov A.A., Mikhalevsky V.A., Eliseev N.N., Kiselev A.V., Burtsev A.A., Ionin V.V., Maliutin A.M., Khmelenin D.N., Glebov V.N., Lotin A.A. Two-stage conductivity switching of GST thin films induced by femtosecond laser radiation // Optics & Laser Technology. 2023. V. 157. P. 108773. https://doi.org/10.1016/j.optlastec.2022.108773
- Ovshinsky S.R. Optical cognitive information processing – a new field // Japanese Journal of Applied Physics. 2004. V. 43. N 7S. P. 4695. https://doi.org/10.1143/JJAP.43.4695
- Huber E., Marinero E.E. Laser-induced crystallization of amorphous GeTe: A time-resolved study // Physical Review B. 1987. V. 36. N 3. P. 1595–1604. https://doi.org/10.1103/PhysRevB.36.1595
- Kiselev A.V., Mikhalevsky V.A., Burtsev A.A., Ionin V.V., Eliseev N.N., Lotin A.A. Transmissivity to reflectivity change delay phenomenon observed in GeTe thin films at laser-induced reamorphization // Optics & Laser Technology. 2021. V. 143. P. 107305. https://doi.org/10.1016/j.optlastec.2021.107305
- Hase M., Mizoguchi K., Nakashima S. Generation of coherent THz phonons in GeTe ferroelectrics // Journal of Luminescence. 2000. V. 87-89. P. 836–839. https://doi.org/10.1016/S0022-2313(99)00433-0
- Nath P., Chopra K.L. Thermal conductivity of amorphous and crystalline Ge and GeTe films // Physical Review B. 1974. V. 10. N 8. P. 3412–3418. https://doi.org/10.1103/PhysRevB.10.3412
- Andrikopoulos K.S., Yannopoulos S.N., Voyiatzis G.A., Kolobov A.V., Ribes M., Tominaga J. Raman scattering study of the a-GeTe structure and possible mechanism for the amorphous to crystal transition // Journal of Physics: Condensed Matter. 2006. V. 18. N 3. P. 965–979. https://doi.org/10.1088/0953-8984/18/3/014
- Burtsev A.A., Ionin V.V., Kiselev A.V., Eliseev N.N., Mikhalevsky V.A., Lotin A.A. Laser-induced crystallization kinetics of GeTe and Ge2Sb2Te5 thin films // Advanced Laser Technologies: Book of abstracts the 28th International Conference. 2021. P. 81. https://doi.org/10.24412/cl-35039-2021-21-81-81
- Lu Q.M., Libera M. Microstructural measurements of amorphous GeTe crystallization by hot‐stage optical microscopy // Journal of Applied Physics. 1995. V. 77. N 2. P. 517–521. https://doi.org/10.1063/1.359034
- Orava J., Greer A.L. Classical-nucleation-theory analysis of priming in chalcogenide phase-change memory // Acta Materialia. 2017. V. 139. P. 226–235. https://doi.org/10.1016/j.actamat.2017.08.013