Menu
Publications
2024
2023
2022
2021
2020
2019
2018
2017
2016
2015
2014
2013
2012
2011
2010
2009
2008
2007
2006
2005
2004
2003
2002
2001
Editor-in-Chief
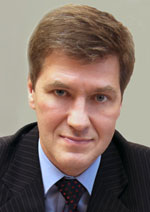
Nikiforov
Vladimir O.
D.Sc., Prof.
Partners
doi: 10.17586/2226-1494-2022-22-5-873-880
Optimization of the optical scheme of a photodetector module operating in the spectral range of 1.3–1.6 μm
Read the full article

Article in Russian
For citation:
Abstract
For citation:
Kovach Y.N., Andryushkin V.V., Kolodeznyi E.S., Novikov I.I., Petrenko A.A., Kamarchuk A.V., Rochas S.S., Bauman D.A. Optimization of the optical scheme of a photodetector module operating in the spectral range of 1.3–1.6 μm. Scientific and Technical Journal of Information Technologies, Mechanics and Optics, 2022, vol. 22, no. 5, pp. 873–880 (in Russian). doi: 10.17586/2226-1494-2022-22-5-873-880
Abstract
Optical system consisting of single-mode optical fiber and p-i-n photodiode semiconductor chip with InGaAs active layer was investigated. Considered photodetector module has responsivity in 1.3–1.6 µm. The problem of optical power loss due to inaccurate matching between the optical fiber and the active medium of photodiode in photodetector modules is investigated; resolving the power loss problem will lead to an increase in the spectral photosensitivity and external quantum efficiency of the photodetector module. Optimization of optical fiber coupling with semiconductor chip was implemented in Zemax® software with built-in Levenberg–Marquardt algorithm. Also, numerical calculations of the influence of the transverse and longitudinal displacement on optical coupling efficiency in the photodetector module were carried out. The optical system of photodetector module based on standard metal can package was built in Zemax® software. Optimal distances between elements of the photodetector module were calculated, and maximum efficiency of 93.1 % optical coupling between single-mode fiber and photodiode aperture was achieved. The necessary sensitivity of linear micro translators used during the assembly of photodetector modules was determined to ensure the alignment of optical elements with coupling efficiency more than 90 %. The results of this work can be used in the design of photodetector modules. The proposed solutions can be relatively easily modified to create photodetector modules of other spectral ranges.
Keywords: optical coupling, photodetector module, optimization, Zemax® software
Acknowledgements. This work was supported by the Program of the Formation and Development of the NTI Center of Excellence in Photonics with the government support of the Foundation for National Technology Initiative’s Project Support and the Ministry of Science and Higher Education of the Russian Federation (grant agreement No. 70-2021-00309 of 12.18.2021; R&D contract No. 763 of 26.04.2022) for the part of optical modeling and photoresponsivity calculation and by Priority 2030 program for the part of heterostructure characterization
References
Acknowledgements. This work was supported by the Program of the Formation and Development of the NTI Center of Excellence in Photonics with the government support of the Foundation for National Technology Initiative’s Project Support and the Ministry of Science and Higher Education of the Russian Federation (grant agreement No. 70-2021-00309 of 12.18.2021; R&D contract No. 763 of 26.04.2022) for the part of optical modeling and photoresponsivity calculation and by Priority 2030 program for the part of heterostructure characterization
References
-
Tekin T. Review of packaging of optoelectronic, photonic, and MEMS component. IEEE Journal on Selected Topics in Quantum Electronics, 2011, vol. 17, no. 3, pp. 704–719. https://doi.org/10.1109/JSTQE.2011.2113171
-
Zimmermann L., Preve G.B., Tekin T., Rosin T., Landles K. Packaging and assembly for integrated photonics – a review of the ePIXpack photonics packaging platform. IEEE Journal on Selected Topics in Quantum Electronics, 2011, vol. 17, no. 3, pp. 645–651. https://doi.org/10.1109/JSTQE.2010.2084992
-
Fischer-Hirchert U.H.P. Photonic Packaging Sourcebook. Springer-Verlag Berlin Heidelberg, 2015, 325 p. https://doi.org/10.1007/978-3-642-25376-8
-
Zaboub M., Guessouma A., Demaghab N.-E., Guermata A. Fabrication of polymer microlenses on single mode optical fibers for light coupling. Optics Communications, 2016, vol. 366, pp. 122–126. https://doi.org/10.1016/j.optcom.2015.12.010
-
Latry O., Ketata M., Ketata K., Debrie R. Optimization of the coupling between a tapered fibre and a p-i-n photodiode. Journal of Physics D: Applied Physics, 1995, vol. 28, no. 8, pp. 1562–1572. https://doi.org/10.1088/0022-3727/28/8/004
-
Sakai K., Kawano M., Aruga H., Takagi S.-I., Kaneko S.-I., Suzuki J., Negishi M., Kondoh Y., Fukuda K.-I. Photodiode packaging technique using ball lens and offset parabolic mirror. Journal of Lightwave Technology, 2009, vol. 27, no. 17, pp. 3874–3879. https://doi.org/10.1109/JLT.2009.2020068
-
Mangal N., Missinne J., Van Campenhout J., Snyder B., Van Steenberge G. Ball lens embedded through-package via to enable backside coupling between silicon photonics interposer and board-level interconnects. Journal of Lightwave Technology, 2020, vol. 38, no. 8, pp. 2360–2369. https://doi.org/10.1109/JLT.2020.2966446
-
Ori T., Masuko K. Bi-directional optical module. Patent US7917036B2, 2011, pp. 20.
-
Wang K.-W., Lin C.-C., Li C.-J., Chang C., Shih T.-T., Chuang Y.-C. Wavelength division multiplexing and demultiplexing transistor outline (TO)-can assemblies for use in optical communications, and methods. Patent US9784919B2, 2017, pp. 15.
-
Baek J.-M., Park J.-W. Bidirectional optical transceiver. Patent US7281865B2, 2007, pp. 13.
-
Ball lans unit for transmitter/receiver optical sub assembly of transceiver, and apparatus and method for manufacturing the same. Patent KR100746260B1, 2007, pp. 12.(in Korean)
-
Blasingame R.W., Chen B.S., Lee J.C., Orenstein J.D., Guenter J.K. Pluggable optical optic system having a lens fiber stop. Patent US7298942B2, 2007, pp. 14.
-
Optical sub-module structure for optical fibre transceiver.Patent CN2607584Y, 2003, pp. 20.(in Chinese)
-
Lu S., Zhang F., Xu C., Duan J. Coupling efficiency of a laser diode to a single-mode fiber via a microlens on the fiber tip. Optical Fiber Technology, 2022, vol. 68, pp. 102766. https://doi.org/10.1016/j.yofte.2021.102766
-
Junhong Y., Linhui G., Hualing W., Huicheneng M., Hao T., Songxin G., Deyong W. Analysis influence of fiber alignment error on laser–diode fiber coupling efficiency. Optik, 2016, vol. 127, no. 6, pp. 3276–3280. https://doi.org/10.1016/j.ijleo.2015.11.219
-
Ramesh R., Tiwari N., Joshi P. Design of a coupling lens assembly and study on the impact of optical misalignments and variations of lens assembly on BER of a system. Proc. of the 2017 International Conference on Nextgen Electronic Technologies: Silicon to Software (ICNETS2), 2017, pp. 10–13. https://doi.org/10.1109/ICNETS2.2017.8067886
-
Yang C.-C., Huang Y.-H., Peng T.-C., Wu M.-C., Ho C.-L., Hong C.-C., Liu I.-M., Tsai Y.-T. Monte Carlo ray trace simulation for micro-ball-lens-integrated high-speed InGaAs p-i-n photodiodes. Journal of Applied Physics, 2007, vol. 101, no. 3, pp. 033107. https://doi.org/10.1063/1.2432484
-
Engelbrecht J.A.A. An assessment of some theoretical models used for the calculation of the refractive index of InXGa1−xAs. Physica B: Condensed Matter, 2018, vol. 535, pp. 8–12. https://doi.org/10.1016/j.physb.2017.05.047
-
Dinges H.W., Burkhard H., Lösch R., Nickel H., Schlapp W. Refractive indices of InAlAs and InGaAs/InP from 250 to 1900 nm determined by spectroscopic ellipsometry. Applied Surface Science, 1992, vol. 54, pp. 477–481. https://doi.org/10.1016/0169-4332(92)90090-K
-
Dinges H.W., Burkhard H., Lösch R., Nickel H., Schlapp W. Determination of refractive indexes of In0.52Al0.48As on InP in the wavelength range from 250 to 1900 nm by spectroscopic ellipsometry. Materials Science and Engineering: B, 1993, vol. 20, no. 1–2, pp. 180–182. https://doi.org/10.1016/0921-5107(93)90423-K
-
Pettit G.D., Turner W.J. Refractive index of InP. Journal of Applied Physics, 1965, vol. 36, no. 6, pp. 2081. https://doi.org/10.1063/1.1714410
-
Luke K., Okawachi Y., Lamont M.R.E., Gaeta A.L., Lipson M. Broadband mid-infrared frequency comb generation in a Si3N4 microresonator. Proc. of the Conference on Lasers and Electro-Optics (CLEO), 2015, pp. 7184257. https://doi.org/10.1364/CLEO_SI.2015.STu4I.8
-
KolodeznyiE.S., NovikovI.I., GladyshevA.G., RochasS.S., SharipoK.D., KarachinskyL.Ya., EgorovA.Yu., BougrovV.E. Studyofantireflectioncoatingsforhigh-speed1.3 -1.55 µmInGaAs/InPPINphotodetector. MaterialsPhysicsandMechanics,2017,vol. 32,no. 2,pp. 194–197. https://doi.org/10.18720/MPM.3222017-11
-
BlistanovA.A.Crystals of Quantum and Nonlinear Optics.Moscow, MISIS Publ., 2000, 432 p. (in Russian)
-
Korte S., Farrer I., Beere H.E., Clegg W.J. Discontinuous yield in InGaAs thin films. Surface and Coatings Technology, 2008, vol. 203, no. 5–7, pp. 713–716. https://doi.org/10.1016/j.surfcoat.2008.08.052
-
Kelly R.L. Program of the 1972 Annual Meeting of the Optical Society of America. Journal of the Optical Society of America, 1972, vol. 62, no. 11, pp. 1336. https://doi.org/10.1364/JOSA.62.001336
-
Kurtash V.A., Egorenkov A.A. Investigation of the optical properties of the photocathode InP/InGaAs/InP structures. Proc. of the XI Annual Scientific and Technical Conference of Young Professionals «Modern photoelectronics technique and technology». Available at: http://www.niielectron.ru/issledovanie-opticheskih-svojstv-struktur-fotokatoda-inp-ingaas-inp/(accessed: 01.09.2022). (in Russian)
-
Zemax User’s Manual. 2014, 879 p.
-
Chen S., Chen J. Optimization of absorption layer in InGaAs/InP uni-traveling carrier photodiode. Proceedings of SPIE, 2021, vol. 11781, pp. 117811E. https://doi.org/10.1117/12.2591305
-
WangX.D., HuW.D., ChenX.S., XuJ.T., LiX.Y., LuW. PhotoresponsestudyofvisibleblindGaN/AlGaNp-i-nultravioletphotodetector. OpticalandQuantumElectronics,2011,vol. 42,no. 11,pp. 755–764. https://doi.org/10.1007/s11082-011-9473-8
-
Rochas S.S., Kolodeznyi E.S., Kozyreva O.A., Voropaev K.O., Sudas D.P., Novikov I.I., Egorov A.Yu. A heterostructure for resonant-cavity GaAs p-i-n photodiode with 840-860 nm wavelength. Journal of Physics: Conference Series, 2019, vol. 1236, no. 1, pp. 012071. https://doi.org/10.1088/1742-6596/1236/1/012071