Menu
Publications
2024
2023
2022
2021
2020
2019
2018
2017
2016
2015
2014
2013
2012
2011
2010
2009
2008
2007
2006
2005
2004
2003
2002
2001
Editor-in-Chief
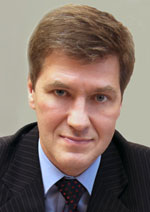
Nikiforov
Vladimir O.
D.Sc., Prof.
Partners
doi: 10.17586/2226-1494-2023-23-1-202-209
Simulation of diffusion processes during electrothermal treatment of reaction crucibles of the Fe-Sn system
Read the full article

Article in Russian
For citation:
Abstract
For citation:
Fomin V.E., Tukmakova A.S., Bolkunov G.A., Novotelnova A.V., Bochkanov F.Yu., Karpenkov D.Yu. Simulation of diffusion processes during electrothermal treatment of reaction crucibles of the Fe-Sn system. Scientific and Technical Journal of Information Technologies, Mechanics and Optics, 2023, vol. 23, no. 1, pp. 202–209 (in Russian). doi: 10.17586/2226-1494-2023-23-1-202-209
Abstract
The diffusion processes regularity in the reaction crucibles of the iron-tin system during their electrothermal treatment was studied by the numerical simulation methods. The effect of current density and temperature on the processes of heat and mass transfer in the reaction zone has been studied. Numerical simulation was performed by the finite element method. The developed model includes mechanical, thermal, electrical and chemical processes during the electrothermal treatment of the iron-tin system in the reaction crucible, taking into account the distribution of components under various processing conditions of the reaction crucible. A comparative analysis of the calculated data on the diffusion of tin into iron under conditions of long-term exposure to high temperatures without the application of an electric voltage and when the reaction zone is heated by passing a high-density electric current is performed. A picture of the distribution of mass fractions of components depending on the type of impact is obtained. The penetration depth of the interacting components was determined and the intensity of the mass transfer processes was assessed. The regularities of heat and mass transfer in the system of iron and tin with a change of the process initial parameters are established. The model was verified by comparing the simulation results with the data of full-scale experiments on control samples. The research results can be used to predict the conditions for obtaining new functional materials.
Keywords: computer simulation, thermal conductivity, electrical conductivity, diffusion, thermal and electric fields
References
References
-
Li X., Xu L., Ding L., Wang J., Shen M., Lu X., Zhu Z., Behnia K. Anomalous Nernst and Righi-Leduc effects in Mn3Sn: Berry curvature and entropy flow. Physical Review Letters, 2017, vol. 119, no. 5, pp. 056601. https://doi.org/10.1103/PhysRevLett.119.056601
-
Bulat L.P., Nefedova I.A. Nonlinear thermoelectric phenomena. Journal of International Academy of Refrigeration, 2012, no. 4, pp. 54–56. (in Russian)
-
Sales B.C., Saparov B., McGuire M.A., Singh D.J., Parker D.S. Ferromagnetism of Fe3Sn and alloys. Scientific Reports, 2014, vol. 4, no. 1, pp. 7024. https://doi.org/10.1038/srep07024
-
Predel B. Fe-Sn (Iron-Tin). Dy-Er–Fr-Mo, 1995, pp. 1–5. https://doi.org/10.1007/10474837_1342
-
Levashov E.A., Mukasyan A.S., Rogachev A.S., Shtansky D.V. Self-propagating high-temperature synthesis of advanced materials and coatings. International Materials Reviews, 2017, vol. 62, no. 4, pp. 203–239. https://doi.org/10.1080/09506608.2016.1243291
-
Orrù R., Licheri R., Locci A.M., Cincotti A., Cao G. Consolidation/synthesis of materials by electric current activated/assisted sintering. Materials Science and Engineering: R: Reports, 2009, vol. 63, no. 4-6, pp. 127–287. https://doi.org/10.1016/j.mser.2008.09.003
-
Nikbakht R., Assadi H. Phase-field modelling of self-propagating high-temperature synthesis of NiAl. Acta Materialia, 2012, vol. 60, no. 10, pp. 4041–4053. https://doi.org/10.1016/j.actamat.2012.04.017
-
Lin S., Yeh C., Xie W., Liu Y., Yoshimura M. Ab initio-aided CALPHAD thermodynamic modeling of the Sn-Pb binary system under current stressing. Scientific Reports, 2013, vol. 3, no. 1, pp. 2731. https://doi.org/10.1038/srep02731
-
Fayyazi B., Skokov K.P., Faske T., Karpenkov D.Y., Donner W., Gutfleisch O. Bulk combinatorial analysis for searching new rare-earth free permanent magnets: Reactive crucible melting applied to the Fe-Sn binary system. Acta Materialia, 2017, vol. 141, pp. 434–443. https://doi.org/10.1016/j.actamat.2017.09.036
-
Chen C.-M., Chen S.-W. Electromigration effect upon the Zn/Ni and Bi/Ni interfacial reactions. Journal of Electronic Materials, 2000, vol. 29, no. 10, pp. 1222–1228. https://doi.org/10.1007/s11664-000-0016-5
-
Pierce D.G., Brusius P.G. Electromigration: A review. Microelectronics Reliability, 1997, vol. 37, no. 7, pp. 1053–1072. https://doi.org/10.1016/s0026-2714(96)00268-5
-
Goll D., Loeffler R., Hohs D., Schneider G. Reaction sintering as a high-throughput approach for magnetic materials development. Scripta Materialia, 2018, vol. 146, pp. 355–361. https://doi.org/10.1016/j.scriptamat.2017.05.004
-
Buch A. Pure Metals Properties: A Scientific and Technical Handbook. ASM International, 1999, 306 p.
-
Iwashita N., Imagawa H., Nishiumi W. Variation of temperature dependence of electrical resistivity with crystal structure of artificial graphite products. Carbon, 2013, vol. 61, pp. 602–608. https://doi.org/10.1016/j.carbon.2013.05.042
-
Patel A.B., Bhatt N.K., Thakore B.Y., Vyas P.R., Jani A.R. The temperature-dependent electrical transport properties of liquid Sn using pseudopotential theory.Molecular Physics, 2014, vol. 112, no. 15, pp. 2000–2004. https://doi.org/10.1080/00268976.2013.877169
-
Klemens P.G., Pedraza D.F. Thermal conductivity of graphite in the basal plane. Carbon, 1994, vol. 32, no. 4, pp. 735–741. https://doi.org/10.1016/0008-6223(94)90096-5
-
Eiling A., Schilling J.S. Pressure and temperature dependence of electrical resistivity of Pb and Sn from 1-300K and 0-10 GPa-use as continuous resistive pressure monitor accurate over wide temperature range; superconductivity under pressure in Pb, Sn and In. Journal of Physics F: Metal Physics, 1981, vol. 11, no. 3, pp. 623–639. https://doi.org/10.1088/0305-4608/11/3/010
-
Chapman T.W. The heat capacity of liquid metals. Materials Science and Engineering, 1966, vol. 1, no. 1, pp. 65–69. https://doi.org/10.1016/0025-5416(66)90012-7
-
Taylor G.R., Isin A., Coleman R.V. Resistivity of iron as a function of temperature and magnetization. Physical Review, 1968, vol. 165, no. 2, pp. 621–631. https://doi.org/10.1103/physrev.165.621
-
Torres D.N., Perez R.A., Dyment F. Diffusion of tin in α-iron. Acta Materialia, 2000, vol. 48, no. 11, pp. 2925–2931. https://doi.org/10.1016/s1359-6454(00)00074-4
-
Neumann G., Tuijn C. Self-Diffusion and Impurity Diffusion in Pure Metals: Handbook of Experimental Data. Elsevier, 2011, 360 p.
-
Ishida T. The reaction of solid iron with molten tin. Transactions of the Japan Institute of Metals, 1973, vol. 14, no. 1, pp. 37–44. https://doi.org/10.2320/matertrans1960.14.37