Menu
Publications
2025
2024
2023
2022
2021
2020
2019
2018
2017
2016
2015
2014
2013
2012
2011
2010
2009
2008
2007
2006
2005
2004
2003
2002
2001
Editor-in-Chief
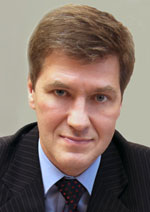
Nikiforov
Vladimir O.
D.Sc., Prof.
Partners
doi: 10.17586/2226-1494-2017-17-6-1153-1158
LOWER LIMB EXOSKELETONS: BRIEF REVIEW
Read the full article

Article in English
For citation: Ergasheva B.I. Lower limb exoskeletons: brief review. Scientific and Technical Journal of Information Technologies, Mechanics and Optics , 2017, vol. 17, no. 6, pp. 1153–1158 (in English). doi: 10.17586/2226-1494-2017-17-6-1153-1158
Abstract
For citation: Ergasheva B.I. Lower limb exoskeletons: brief review. Scientific and Technical Journal of Information Technologies, Mechanics and Optics , 2017, vol. 17, no. 6, pp. 1153–1158 (in English). doi: 10.17586/2226-1494-2017-17-6-1153-1158
Abstract
The paper provides a brief review on structural and technological features of Lower Limb Exoskeletons that have been manufactured until recently and the description of their disadvantages. Exoskeleton is a device designed to compensate for the lost functions of a human operator by increasing muscle strength and expanding movement amplitude with its outer frame and driving parts. Lower Limb Exoskeletons are developed to support people who have partially or completely lost lower limbs dynamics. The research and development background dates back to 1960s. Over the years, great progress has been made by scientists and researchers from all around the world. However, despite various strategies and attempts to achieve perfection in operating an exoskeleton in the current state of science and technology, it is still a challenge to develop an auxiliary model that endows with both super-efficiency and naturalness. Consequently, the paper intends to highlight the problems to be resolved and the future trends in this field. Exoskeletons have been limited in their availability for wider application by general population because of their high cost. Moreover, technological and structural issues related to design, safety, framework deterioration and optimization remain open-ended. As a technological breakthrough is an evolving process, this review can assist in conducting current research and making recommendations for perspective developments in the field of Lower Limb Exoskeletons.
Keywords: exoskeleton, lower limb exoskeleton, robotics, robots, review, rehabilitation, legs, orthosis, assistive device
References
References
1. Vukobratovic M.K. When were active exoskeletons actually born? // International Journal of Humanoid Robotics. 2007.
V. 4. N 3. P. 459–486. doi: 10.1142/S0219843607001163
2. Vukobratovic M.K. Active exoskeletal systems and beginning of the development of humanoid robotic / In: Monograph of ANS: Academy of Nonlinear Sciences. Advances in Nonlinear Sciences II – Sciences and Applications. Belgrade, 2008. V. 2. P. 329–348.
3. Vukobratovic M.K., Hristic D., Stojiljkovic Z. Development of active anthropomorphic exoskeletons // Medical and Biological Engineering. 1974. V. 12. N 1. P. 66–80. doi:10.1007/BF02629836
4. Hristic D., Vukobratovic M.K. Active exoskeletons future rehabilitation aids for severely handicapped persons // Orthopedie Technique. 1976. N 19. P. 221–224.
5. Vukobratovic M.K., Borovac B., Stokic D., Surdilovic D. Humanoid robots / In: Mechanical Systems Design Handbook: Modeling, Measure and Control. CRC Press, 2001. P. 727–777. doi:
6. Kazerooni H. Human augmentation and exoskeleton systems in Berkeley // International Journal of Humanoid Robotics. 2007. V. 4. N 3. P. 575–605. doi: 10.1142/S0219843607001187
7. Wang S., Wang L., Meijneke C., van Asseldonk E., Hoellinger T. et al. Design and control of the MINDWALKER exoskeleton // IEEE Transactions on Neural Systems and Rehabilitation Engineering. 2015. V. 23. N 2. P. 277–286. doi: 10.1109/TNSRE.2014.2365697
8. Wang L., Wang S., Edwin H.F. van Asseldonk E., van der Kooij H. Actively controlled lateral gait assistance in a lower limb exoskeleton // Proc. IEEE Conf. on Intelligent Robots and Systems (IROS). Tokyo, Japan, 2013. P. 965–970. doi: 10.1109/IROS.2013.6696467
9. Wang S., Meijneke C., van der Kooij H. Modeling, design, and optimization of Mindwalker series elastic joint // IEEE 13th Int. Conf. on Rehabilitation Robotics. Seattle, USA, 2013. doi:10.1109/ICORR.2013.6650381
10. Krut S., Benoit M., Dombre E., Pierrot F. MoonWalker, a lower limb exoskeleton able to sustain bodyweight using a passive force balancer // Proc. IEEE Int. Conf. on Robotics and Automation. Anchorage, USA, 2010. P. 2215–2220. doi: 10.1109/ROBOT.2010.5509961
11. Park Y.-L., Chen B.R., Perez-Arancibia N.O. et al. Design and control of a bio-inspired soft wearable robotic device for ankle, foot rehabilitation // Bioinspiration and Biomimetics. 2014.
V. 9. N 1. doi: 10.1088/1748-3182/9/1/016007
V. 9. N 1. doi: 10.1088/1748-3182/9/1/016007
12. Farris D.J., Hicks J.L., Delp S.L., Sawicki G.S. Musculoskeletal modelling deconstructs the paradoxical effects of elastic ankle exoskeletons on plantar-flexor mechanics and energetics during hopping // Journal of Experimental Biology. 2014. V. 217.
N 22. P. 4018–4028. doi:10.1242/jeb.107656
N 22. P. 4018–4028. doi:10.1242/jeb.107656
13. Curtis S., Kobetic R., Bulea T.C. et al. Sensor-based hip control with hybrid neuroprosthesis for walking in paraplegia // Journal of Rehabilitation Research and Development. 2014. V. 51. N 2. P. 229–244. doi: 10.1682/JRRD.2012.10.0190
14. Murray S.A., Ha K.H., Hartigan C., Goldfarb M. An assistive control approach for a lower-limb exoskeleton to facilitate recovery of walking following stroke // IEEE Transactions on Neural Systems and Rehabilitation Engineering. 2014. V. 23.
N 3. P. 441–449. doi: 10.1109/TNSRE.2014.2346193
N 3. P. 441–449. doi: 10.1109/TNSRE.2014.2346193
15. Del-Ama A.J., Gil-Agudo A., Pons J.L., Moreno J.C. Hybrid FES-robot cooperative control of ambulatory gait rehabilitation exoskeleton // Journal of Neuro Engineering and Rehabilitation. 2014. V. 11. N 1. Art. 27. doi: 10.1186/1743-0003-11-27
16. Cruciger O., Schildhauer T.A., Meindl R.C. et. al. Impact of locomotion training with a neurologic controlled hybrid assistive limb (HAL) exoskeleton on neuropathic pain and health related quality of life (HRQoL) in chronic SCI: a case study // Disability and Rehabilitation: Assistive Technology. 2014. V. 11. N 6. P. 529–534. doi:10.3109/17483107.2014.981875
17. Van Dijk W., Van der Kooij H. Optimization of human walking for exoskeletal support // Proc. IEEE Int. Conf. on Rehabilitation Robotics. Seattle, USA, 2013. doi:10.1109/ICORR.2013.6650394
18. Kazerooni H. The human power amplifier technology at the University of California, Berkeley // Robotics and Autonomous Systems. 1996. V. 19. N 2. P. 179–187.
19. Banala S.K., Agrawal S.K., Scholz J.P. Active Leg Exoskeleton (ALEX) for gait rehabilitation of motor-impaired patients // Proc. IEEE 10th Int. Conf. on Rehabilitation Robotics. Noordwijk, Netherlands, 2007. P. 401–407. doi: 10.1109/ICORR.2007.4428456
20. Banala S.K., Kim S.H, Agrawal S.K., Scholz J.P. Robot assisted gait training with Active Leg Exoskeleton (ALEX) // IEEE Transactions on Neural Systems and Rehabilitation Engineering. 2009. V. 17. N 1. P. 2–8. doi: 10.1109/TNSRE.2008.2008280
21. Banala S.K., Agrawal S.K., Kim S.H., Scholz J.P. Novel gait adaptation and neuromotor training results using an active leg exoskeleton // IEEE/ASME Transactions on Mechatronics. 2010. V. 15. N 2. P. 216–225. doi: 10.1109/TMECH.2010.2041245
22. Okubo A., Kiyama T., Osuka K., Shirogauchi G., Oya R., Fujimoto H. A dynamic model of power-assistive machinery with high strength-amplification // Proceedings of SICE Annual Conference. 2010. P. 2026–2029.
23. Rosen J., Arcan M. Performances of hill-type and neural network muscle models – towards a myosignal based exoskeleton // Computers and Biomedical Research. 1999. V. 32. N 5. P. 415–439. doi: 10.1006/cbmr.1999.1524
24. Harada K., Kajita S., Kanehiro F. et al. Real-time planning of humanoid robot's gait for force-controlled manipulation // IEEE/ASME Transactions on Mechatronics. 2007. V. 12. N 1. P. 53–62. doi: 10.1109/TMECH.2006.886254
25. Kajita S., Morisawa M., Miura K. et al. Biped walking stabilization based on linear inverted pendulum tracking // Proc. IEEE/RSJ Int. Conf. on Intelligent Robots and Systems (IROS). Taipei, Taiwan, 2010. P. 4489–4496. doi: 10.1109/IROS.2010.5651082
26. Sellaouti R., Stasse O., Kajita S. et al. Faster and smoother walking of humanoid HRP-2 with passive toe joints // Proc. IEEE/RSJ Int. Conf. on Intelligent Robots and Systems (IROS). Beijing, China, 2006. P. 4909–4914. doi: 10.1109/IROS.2006.282449
27. Arisumi H., Miossec S., Chardonnet J.R. Dynamic lifting by whole body motion of humanoid robots // Proc. IEEE/RSJ Int. Conf. on Intelligent Robots and Systems (IROS). Nice, France, 2008. P. 668–678. doi:10.1109/IROS.2008.4651195
28. Talaty M., Esquenazi A., Briceno J.E. Differentiating ability in users of the ReWalkTM powered exoskeleton: an analysis of walking kinematics // Proc. IEEE 13th Int. Conf. on Rehabilitation Robotics. Seattle, USA, 2013. doi: 10.1109/ICORR.2013.6650469
29. Ryder M.C., Sup F. Leveraging gait dynamics to improve efficiency and performance of powered hip exoskeletons // Proc. IEEE 13th Int. Conf. on Rehabilitation Robotics. Seattle, USA, 2013. doi: 10.1109/ICORR.2013.6650440
30. Elliott G., Sawicki G.S., Marecki A., Herr H. The biomechanics and energetics of human running using an elastic knee exoskeleton // Proc. IEEE 13th Int. Conf. on Rehabilitation Robotics. Seattle, USA, 2013. doi: 10.1109/ICORR.2013.6650418
31. Колюбин С.А., Мусалимов В.М. Биомехатроника: шаги навстречу энергоэффективным роботам // ControlEngineeringРоссия. 2017. № 2(68). С. 92–95.
32. Hassan M., Kadone H., Suzuki K., Sankai Y. Wearable gait measurement system with an instrumented cane for exoskeleton control // Sensors. 2014. V. 14. N 1. P. 1705–1722. doi:10.3390/s140101705
33. Боровин Г.К., Костюк А.В., Сит Д. Компьютерное моделирование гидравлической системы управления экзоскелетона // Препринты ИПМ им. М.В. Келдыша. 2004. № 79. С. 1–24.
34. Meuleman J., Van Asseldonk E.H.F., Van Der Kooij H. Novel actuation design of a gait trainer with shadow leg approach // Proc. IEEE 13th Int. Conf. on Rehabilitation Robotics. Seattle, USA, 2013. doi: 10.1109/ICORR.2013.6650369
35. Sylos-Labini F., La Scaleia V., d’Avella A., et al. EMG patterns during assisted walking in the exoskeleton // Frontiers in Human Neuroscience. 2014. V. 8. Art. 423. doi: 10.3389/fnhum.2014.00423
36. Yu H., Huang S., Thakor N.V. et al. A novel compact compliant actuator design for rehabilitation robots // Proc. IEEE 13th Int. Conf. on Rehabilitation Robotics. Seattle, USA, 2013. doi: 10.1109/ICORR.2013.6650478
37. Yan T., Cempini M., Oddo C.M., Vitiello N. Review of assistive strategies in powered lower-limb orthoses and exoskeletons // Robotics and Autonomous System. 2015. V. 64. P. 120–136. doi: 10.1016/j.robot.2014.09.032
38. Hyun D.J., Park H., Ha T., Park S., Jung K. Biomechanical design of an agile, electricity-powered lower-limb exoskeleton for weight-bearing assistance // Robotics and Autonomous Systems. 2017. V. 95. P. 181–195. doi: 10.1016/j.robot.2017.06.010
39. Aliman N., Ramli R., Haris S.M. Design and development of lower limb exoskeletons: a survey // Robotics and Autonomous Systems. 2017. V. 95. P. 102–116. doi: 10.1016/j.robot.2017.05.013
40. Dollar A.M., Herr H. Design of a quasi-passive knee exoskeleton to assist running // Proc. IEEE/RSJ Int. Conf. on Intelligent Robots and Systems (IROS). Nice, France, 2008. P. 747–754. doi: 10.1109/IROS.2008.4651202