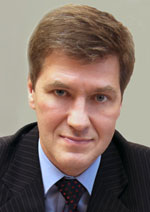
Nikiforov
Vladimir O.
D.Sc., Prof.
doi: 10.17586/2226-1494-2019-19-4-567-573
RESEARCHOF MAGNETIC RESONANCE QUALITY FACTOR DEPENDENCE ON CESIUM DISTRIBUTION IN QUANTUM ROTATION SENSOR CELL
Read the full article

For citation:
Abstract
Subject of Research. The paper presents results of the experimental and theoretical studies on cesium distribution effect along the vapor cell walls of quantum rotation sensor on the quality of parametric resonance in cesium. Methods. Theoretical and experimental studies were performed using the mathematical model, quantum rotation sensor layout and the laboratory setup for the vapor cell parameters research. The mathematical model was used for calculation of cesium relaxation rate theoretical value at various temperatures and further comparison of these data with experimental ones. For the experimental determination of cesium relaxation rate, the data on the parametric resonance width in cesium obtained on the of the quantum rotation sensor model were used. Studies of the cell optical properties were carried out on a laboratory setup in non-resonant light. A laser with a vertical emitter similar to the one used in the quantum rotation sensor is applied as a source of non-resonant light, tuned away from the nominal wavelength. The optimal detuning of the optical radiation frequency from the cesium resonance was determined on the laboratory setup, that reduces the dependence of the measurement results on the cell temperature. Selection of laser radiation optimal parameters is performed for the normal mode of the laser operation and freedom from fluctuations in its power and polarization. Main Results. During the research it was shown that undesirable cesium redistribution in the vapor cell leads to the optical paths transparency change, which causes the deterioration of the signal-to-noise ratio and the decrease in the resonance line quality factor. In addition, it was shown that cesium resonance line broadening due to sedimentation of the cesium vapor on the cell walls is insignificant and is at the level of the measurement error. Practical Relevance. We have proposed and tested non-resonant light intensity control method for the laser emitter after passing through a vapor cell. This method provides for an operative state monitoring of the optical pumping channels and detecting of quantum rotation sensor layout.
Acknowledgements. The authors express their appreciation to Gleb V. Bezmen, the supervisor, and other colleagues for research assistance.
References
-
Peshekhonov V.G. Gyroscopic systems: current status and prospects. Gyroscopy and Navigation, 2011, vol. 2, no. 3, pp. 111–118. doi: 10.1134/S2075108711030096
-
Fang J.C., Qin J. Advances in atomic gyroscopes: a view from inertial navigation applications. Sensors, 2012, vol. 12, no. 5, pp. 6331–6346. doi: 10.3390/s120506331
-
Feng D.Review of quantum navigation. IOP Conf. Series: Earth and Environmental Science, 2019, vol. 237, p. 032027. doi: 10.1088/1755-1315/237/3/032027
-
Simpson J.H., Fraser J.T., Greenwood I.A. An optically pumped nuclear magnetic resonance gyroscope. IEEE Transactions on Aerospace, 1963, vol. 1, no. 2, pp. 1107–1110. doi: 10.1109/ta.1963.4319483
-
Larsen M., Bulatowicz M. Nuclear magnetic resonance gyroscope: for DARPA’s micro-technology for positioning, navigation and timing program. Proc. IEEE Int. Frequency Control Symposium, 2012. doi: 10.1109/fcs.2012.6243606
-
Vershovskii A.K., Litmanovich Yu.A., Pazgalev A.S., Peshekhonov V.G. Nuclear magnetic resonance gyro: threshold characteristics. Gyroscopy and Navigation, 2018. vol. 26, no. 1, pp. 55–80. (in Russian) doi: 10.17285/0869-7035.2018.26.1.055-080
-
Meyer D., Larsen M. Nuclear magnetic resonance gyro for inertial navigation. Gyroscopy and Navigation, 2014, no. 1, pp. 3–13. (in Russian)
-
Umarkhodzhaev R.M., Pavlov Yu.V., Vasilyev A.N. History of NMR gyroscope development in Russia in 1960-2000s. Gyroscopy and Navigation, 2018, vol. 26, no. 1, pp. 3–27. (in Russian) doi: 10.17285/0869-7035.2018.26.1.003-027
-
Popov E.N., Barantsev K.A., Litvinov A.N., Kuraptsev A.S., Voskoboinikov S.P., Ustinov S.M., Larionov N.V., Liokumovich L.B., Ushakov N.A., Shevchenko A.N. Frequency line of nuclear magnetic resonance in quantum rotation sensor: negative effect of detection circuit. Gyroscopy and Navigation, 2016, vol. 24, no. 4,pp. 3–13. (in Russian) doi: 10.17285/0869-7035.2016.24.4.003-013
-
Litmanovich Yu.A., Vershovskii A.K., Peshekhonov V.G. Gyroscope based on the effect of nuclear magnetic resonance: past, present, future. Proc. 7th Russian Multiconference on Control Problems, Plenary Meeting. St. Petersburg, 2014, pp. 35–42. (in Russian)
-
Popov E.N., Barantsev K.A., Ushakov N.A., Litvinov A.N., Liokumovich L.B., Shevchenko A.N., Slyarov F.V., Medvedev A.V. Behavior of signal from optical circuit of quantum rotation sensor based on nuclear magnetic resonance.Gyroscopy and Navigation, 2018, vol. 26, no. 1, pp. 93–106. (in Russian) doi: 10.17285/0869-7035.2018.26.1.093-106
-
Walker T.G., Larsen M.S. Spin-exchange-pumped NMR gyros. Advances in Atomic, Molecular, and Optical Physics, 2016, vol. 65. pp. 377–405. doi: 10.1016/bs.aamop.2016.04.002
-
Shevchenko A.N., Kuzmin A.G., Titov Yu.A. Mass-spectrometric measurement of the gas mixtures composition in the cells of a quantum rotate sensor. Scientific Instrument Engineering, 2015, vol. 28, no. 2, pp. 62–68. (in Russian)
-
Kislitsina E.A., Shevchenko A.N. Magnetic field gradient requirements determination to identify a nuclear magnetic resonance gyroscope metrological characteristics. Almanac of the ITMO University Young Scientists Scientific Papers. St. Petersburg, 2018, pp. 176–179. (in Russian)
-
Vershovskii A.K, Pazgalev A.S. Optimization of the Q factor of the magnetic Mx resonance under optical pump conditions. Technical Physics, 2008, vol. 53, no. 5, pp. 646–654. doi: 10.1134/S1063784208050198
-
Pomerantsev N.M., Ryzhkov V.M., Skrotsky G.V. Physical Fundamentals of Quantum Magnetometry. Moscow, Nauka Publ., 1972, 448 p. (in Russian)
-
Maleev N.A., Blokhin S.A., Bobrov M.V., Kuzmenkov A.G., Kulagina M.M., Ustinov V.M. Laser source for compact nuclear magnetic resonance gyroscope. Gyroscopy and Navigation, 2018, vol. 26, no. 1, pp. 81–92.(in Russian) doi: 10.17285/0869-7035.2018.26.1.081-092