Menu
Publications
2025
2024
2023
2022
2021
2020
2019
2018
2017
2016
2015
2014
2013
2012
2011
2010
2009
2008
2007
2006
2005
2004
2003
2002
2001
Editor-in-Chief
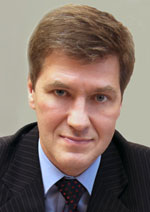
Nikiforov
Vladimir O.
D.Sc., Prof.
Partners
doi: 10.17586/2226-1494-2020-20-2-277-282
СALCULATION OF THERMOPHYSICAL PROPERTIES OF SLAB LASER MULTI-COMPONENT GAS MEDIUM
Read the full article

Article in Russian
For citation:
Abstract
For citation:
Gorbunova A.Yu., Korablev V.A., Zarichnyak Yu.P. Сalculation of thermophysical properties of slab laser multi- component gas medium. Scientific and Technical Journal of Information Technologies, Mechanics and Optics, 2020, vol. 20, no. 2, pp. 277–282 (in Russian). doi: 10.17586/2226-1494-2020-20-2-277-282
Abstract
Subject of Research. The paper presents a method for calculation of thermal conductivity and viscosity of the gaseous slab laser medium. The method is based on the use of the known properties of its components. Thermal conductivity determination is necessary for mathematical and thermal modeling in the process of thermal mode development. The main feature of the proposed technique is application versatility and the possibility to obtain effective characteristics with sufficient accuracy for complex composition gases containing more than two components. Method. The calculation approach lies in consistent application of the Lindsay-Bromley and Wilke formulas for binary gas to the components of the mixture depending on their molar concentration. The component selection order is due to the smallest variance of the calculated values from the reference data. Main Results. The method was verified by comparison of the calculated and experimental data for three-component mixtures. It was found that viscosity measurement error does not exceed 1.5 % with an average deviation of less than 1 %. The average deviation of the values in the case of thermal conductivity is much higher, but the measurement error does not exceed 5 %. For the most frequently used composition of CO2, N2, Xe, and He mixture as a slab laser medium, the thermal properties were calculated for the minimum and maximum concentrations of carbon monoxide arising during operation. The tables show step-by-step application of the method and the dependence of thermal conductivity on temperature. There is a strong increase in thermal conductivity in the range of operating temperatures (more than twice). For example, at room-temperature range (300 K) it is equal to 0.067 W/(m•K), at 800 K it reaches 0.137 W/(m•K). This difference creates a significant non-uniformity of gas medium temperature field and affects drastically the laser stability. Practical Relevance. The results obtained can find practical application in the development of slab lasers and can be used to eliminate power drops and stabilize the operation of existing lasers providing the regulating process for the component concentrations of the gas composition.
Keywords: thermal conductivity, viscosity, multicomponent gas mixture, slab laser, thermal conductivity measurement technique
References
References
-
Brettshnader K. Laser metal working as a key to contract producers success. Photonics Russia, 2009,no. 2,pp. 6–9.(in Russian)
-
Kazakevich V., Yaresko S. Tendencies of development of the market of laser technologies for the solution of problems of laser processing of materials. Part 1. The world laser market. Izvestia of Samara Scientific Center of the Russian Academy of Sciences, 2014,vol. 16, no. 4,pp. 266–275.(in Russian)
-
GorbunovA.K., JdanovS.M., PchelincevaN.I. About possible usage of coppern anopartic lesforinc reasing of durability of sealed CO2-laser. Science and Education, 2012,no. 2, pp. 52. (in Russian)
-
Toptan A. A Novel Approach to Improve Transient Fuel Performance Modeling in Multi-Physics Calculations. North Carolina State University, 2019,157 p.
-
Reid R.C., Prausnitz J.M., Sherwood T.K. The Properties of Gases and Liquids. New York, 1977.
-
Wassiljewa A. Heat conduction in gas mixtures. Physikalische Zeitschrift, 1904, vol. 5, no. 22, pp. 737–742.
-
Lilliestråle A.,Mølnvik M.J., Tangen G., Jakobsen J.P., Munkejord S.T., Morin A., Størset S.O. The IMPACTS Project: The Impact of the Quality of CO2 on Transport and Storage Behaviour. Energy Procedia, 2014, vol. 51, pp. 402–410. doi: 10.1016/j.egypro.2014.07.047
-
Mason E.A., Saxena S.C. Approximate formula for the thermal conductivity of gas mixtures. Physics of Fluids, 1958, vol. 1, no. 5, pp. 361–369. doi: 10.1063/1.1724352
-
Lindsay A.L., Bromley L.A. Thermal conductivity of gas mixtures. Industrial and Engineering Chemistry, 1950, vol. 42, no. 8, pp. 1508–1511. doi: 10.1021/ie50488a017
-
Brokaw R.S. Estimating thermal conductivities fornonpolargas mixtures. Industrial and Engineering Chemistry,1955,vol. 47,no. 11,pp. 2398–2400. doi: 10.1021/ie50551a056
-
Shashkov A.G., Abramenko T.N. Thermal Conductivity of Gas Mixtures. Moscow, EnergyPubl., 1970, 288 p. (in Russian)
-
Singh K., Sood N.K. Viscosity and thermal conductivity of gasmixtures. Indian Journal of Pure& Applied Physics, 2003,vol. 41,no. 2,pp. 121–127.
-
Vargaftik N.B. Handbookon Thermophysical Properties of Gasesand Liquids.Moscow, NaukaPubl., 1972, 720 p. (inRussian)
-
Tsirelman N.M., Komarov A.V. Determination thermophysical properties of multicomponent gas mixtures. Vestnik USATU, 2017,vol. 21, no. 1,pp. 128–135.(in Russian)
-
PerfilevV.O., Skril Y.V., Shushuev D.A. Experimental install at ion of the CO2-laser with slot-holegeometry. Izvestiya of Altai State University, 2005,no. 1,pp. 134–135.(in Russian)
-
Vesnov I.G., Mol'kov S.I., Stepanov V.A., Shishkanov E.F. Dissociation of carbon dioxide in sealed-off CO2 waveguide lasers with radio-frequency excitation. Quantum Electronics, 2000, vol. 30, no. 1, pp. 15–19. doi: 10.1070/QE2000v030n01ABEH001649