Menu
Publications
2024
2023
2022
2021
2020
2019
2018
2017
2016
2015
2014
2013
2012
2011
2010
2009
2008
2007
2006
2005
2004
2003
2002
2001
Editor-in-Chief
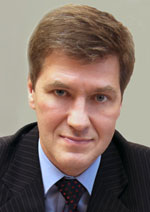
Nikiforov
Vladimir O.
D.Sc., Prof.
Partners
doi: 10.17586/2226-1494-2020-20-3-346-352
FIBER OPTIC MEASUREMENT SYSTEM FOR DETERMINATION OF EXTENDED OBJECT POSITION AND BENDS IN 3D SPACE
Read the full article

Article in Russian
For citation:
Abstract
For citation:
Egorova D.A., Kulikov A.V., Mukhtubaev A.B., Plotnikov M.Yu. Fiber optic measurement system for determination of extended object position and bends in 3D space. Scientific and Technical Journal of Information Technologies, Mechanics and Optics, 2020, vol. 20, no. 3, pp. 346–352 (in Russian). doi: 10.17586/2226-1494-2020-20-3-346-352
Abstract
Subject of Research. Fiber optic measurement systems are widely used in various industries. Most of these systems are fiber optic devices for detecting physical quantities. The development and creation of a measurement system for determination of the location and bends of extended objects in 3D space are actual at the moment. We propose the implementation of the sensitive part of the fiber optic measurement system based on seven single-mode fibers with an array of fiber Bragg gratings fixed to each other. Method. The method for determining the position and bends of extended objects in space is based on finding the level of axial deformation (compression, tension) of the fiber Bragg gratings during bending, and provides the calculation of the curvature direction and magnitude. Further reconstruction of the curve in space is realized by solving a system of differential equations with given initial conditions containing Frenet-Serre formulas. Main Results. The paper presents experiment results on writing fiber Bragg grating arrays into single-mode optical fibers, taking into account the features of the optical scheme and the source spectrum. The design and prototype of the fiber optic measurement system are developed. The results on the reconstruction of the shape based on experimental data are obtained. Practical Relevance. A special feature of this system is the fiber Bragg grating arrays formed in a wide range of wavelengths. Each Bragg grating corresponds to its own reflection wavelength, providing a sensitive part 1 m long. The geometry of the structure is based on seven fiber-optic armored microcables twisted with a given lay length during manufacturing, and provides the measurement of the kink value and direction.
Keywords: optical fiber, fiber Bragg grating array, fiber optic measurement system
Acknowledgements. Personal thanks are expressed to S.V. Varzhel, Head of fiber Bragg grating inscription laboratory of ITMO University, and his scientific team for their help with preparing samples for the prototype.
References
Acknowledgements. Personal thanks are expressed to S.V. Varzhel, Head of fiber Bragg grating inscription laboratory of ITMO University, and his scientific team for their help with preparing samples for the prototype.
References
-
Korotaev V.V., Pantiushin A.V., Serikova M.G., Anisimov A.G. Deflection measuring system for floating dry docks. Ocean Engineering, 2016, vol. 117, pp. 39–44. doi: 10.1016/j.oceaneng.2016.03.012
-
Phillips T., Guenther N., McAree P.R. When the Dust Settles: the four behaviors of LiDAR in the Presence of Fine Airborne Particulates. Journal of Field Robotics, 2017, vol. 34, no. 5, pp. 985–1009. doi: 10.1002/rob.21701
-
Phillips T., Hahn M., McAree R. An evaluation of ranging sensor performance for mining automation applications. Proc. IEEE/ASME International Conference on Advanced Intelligent Mechatronics: Mechatronics for Human Wellbeing (AIM 2013), 2013, pp. 1284–1289. doi: 10.1109/AIM.2013.6584271
-
Dementyev A., Kao H.L.C., Paradiso J.A. SensorTape: modular and programmable 3D-aware dense sensor network on a tape. Proc. 28th Annual ACM Symposium on User Interface Software and Technology, Charlotte, NC, USA, 2015, pp. 649–658. doi: 10.1145/2807442.2807507
-
Danisch L., Chrzanowski A., Bond J., Bazanowski M. Fusion of geodetic and MEMS sensors for integrated monitoring and analysis of deformations. Proc. 13th FIG International Symposium on Deformation Measurements and Analysis, Lisbon, Portugal, 2008, pp. 12–15.
-
Mitrofanova T.A., Vuyko V.V., Zdragewskij R.A., Potahin S.N. Hardware-software complex for the task of spatial position of tools at surgical operations. Vestnik SSTU, 2010, vol. 4, no. 2(50), pp. 112–115. (in Russian)
-
Plamondon A., Delisle A., Larue C., Brouillette D., McFadden D., Desjardins P., Larivière C. Evaluation of a hybrid system for three-dimensional measurement of trunk posture in motion. Applied Ergonomics, 2007, vol. 38, no. 6, pp. 697–712. doi: 10.1016/j.apergo.2006.12.006
-
Parent F., Mandal K.K., Loranger S., Fernandes E.H.W., Kashyap R., Kadoury S. 3D shape tracking of minimally invasive medical instruments using optical frequency domain reflectometry. Proceedings of SPIE, 2016, vol. 9786, pp. 97862J. doi: 10.1117/12.2214998
-
Ledermann Ch., Hergenhan J., Weede O., Woern H. Combining shape sensor and haptic sensors for highly flexible single port system using Fiber Bragg sensor technology. Proc. 8th IEEE/ASME International Conference on Mechatronic and Embedded Systems and Applications (MESA 2012), 2012, pp. 196–201. doi: 10.1109/MESA.2012.627556
-
Pak C.-G. Wing shape sensing from measured strain. AIAA Journal, 2016, vol. 54, no. 3, pp. 1064–1073. doi: 10.2514/1.J053986
-
Lally E., Reaves M., Horrell E., Klute S., Froggatt M.E. Fiber optic shape sensing for monitoring of flexible structures. Proceedings of SPIE, 2012, vol. 8345, pp. 83452Y. doi: 10.1117/12.917490
-
Butov O.V., Bazakutca A.P., Chamorovskii Yu.K., Fedorov A.N., Shevtcov I.A. Wholly fiber-based highly sensitive bending sensor for nuclear industry. Foton-Express, 2019, no. 6(158), pp. 26–27. (in Russian). doi: 10.24411/2308-6920-2019-16008
-
Park Y.-L., Elayaperumal S., Daniel B., Ryu S.C., Shin M., Savall J., Black R.J., Moslehi B., Cutkosky M.R. Real-time estimation of 3-D needle shape and deflection for MRI-guided interventions. IEEE/ASME Transactions on Mechatronics, 2010, vol. 15, no. 6, pp. 906–915. doi: 10.1109/TMECH.2010.2080360
-
Moore J., Rogge M. Shape sensing using multi-core fiber optic cable and parametric curve solutions. Optics Express, 2012, vol. 20, no. 3, pp. 2967–2973. doi: 10.1364/OE.20.002967
-
Egorova D.A., Kulikov A.V., Nikitenko A.N., Gribaev A.I., Varzhel S.V. Investigation of bending effects in chirped FBGs array in multicore fiber. Optical and Quantum Electronics, 2020, vol. 52, no. 2, pp. 130. doi: 10.1007/s11082-020-2251-8
-
Nishio M., Mizutani T., Taked N. Shape identification of variously-deformed composite laminates using Brillouin type distributed strain sensing system with embedded optical fibers. Proceedings of SPIE, 2008, vol. 6932, pp. 69322P. doi: 10.1117/12.775671
-
Duncan R.G., Froggatt M.E., Kreger S.T., Seeley R.J., Gifford D.K., Sang A.K., Wolfe M.S. High-accuracy fiber-optic shape sensing. Proceedings of SPIE, 2007, vol. 6530, pp. 65301S. doi: 10.1117/12.720914
-
Gribaev A.I., Pavlishin I.V., Stam A.M., Idrisov R.F., Varzhel S.V., Konnov K.A. Laboratory setup for fiber Bragg gratings inscription based on Talbot interferometer. Optical and Quantum Electronics, 2016, vol. 48, no. 12, pp. 540. doi: 10.1007/s11082-016-0816-3
-
Kashyap R. Fiber Bragg Gratings. 2nd ed. Elsevier, 2010. 632 p. doi: 10.1016/C2009-0-16830-7
-
Othonos A. Fiber Bragg gratings. Review of Scientific Instruments, 1997, vol. 68, no. 12, pp. 4309–4341. doi: 10.1063/1.1148392
-
Roesthuis R.J., Janssen S., Misra S. On using an array of fiber Bragg grating sensors for closed-loop control of flexible minimally invasive surgical instruments. Proc. 26th IEEE/RSJ International Conference on Intelligent Robots and Systems: New Horizon (IROS 2013), 2013, pp. 2545–2551. doi:10.1109/IROS.2013.6696715
-
Moore J.P. Shape sensing using multi-core fiber. Proc. 2015 Optical Fiber Communications Conference and Exhibition (OFC), 2015, pp. 7121578. doi: 10.1364/OFC.2015.Th1C.2