Menu
Publications
2025
2024
2023
2022
2021
2020
2019
2018
2017
2016
2015
2014
2013
2012
2011
2010
2009
2008
2007
2006
2005
2004
2003
2002
2001
Editor-in-Chief
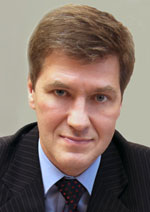
Nikiforov
Vladimir O.
D.Sc., Prof.
Partners
doi: 10.17586/2226-1494-2021-21-1-24-30
ON THE CHOICE OF THE APERTURE DIAMETER OF THE PROBE LASER IN GROUND-BASED ADAPTIVE OPTOELECTRONIC SYSTEMS IN THE FORMATION OF A SODIUM REFERENCE STAR
Read the full article

Article in Russian
For citation:
Abstract
For citation:
Kleymionov V.V., Novikova E.V., Oleynikov M.I. On the choice of the aperture diameter of the probe laser in ground-based adaptive optoelectronic systems in the formation of a sodium reference star. Scientific and Technical Journal of Information Technologies, Mechanics and Optics, 2021, vol. 21, no. 1, pp. 24–30 (in Russian). doi: 10.17586/2226-1494-2021-21-1-24-30
Abstract
Subject of study. The authors propose an integrated approach to the problem of determining the aperture diameter of a probe laser that forms a laser reference star used in ground-based adaptive optoelectronic systems. The relevance of this study is due to the fact that modern large-aperture optical systems for tracking natural stars and artificial objects (spacecraft or fragments of space debris) widely implement the technology of forming laser stars and use them as reference sources for correcting phase distortions of a turbulent atmosphere. The choice of the energy and spatiotemporal characteristics of laser guide stars is related both to the parameters of the probe laser (radiation power and aperture diameter), which forms the guide star, and to the spatiotemporal characteristics of the atmosphere. Method. The diameter of the probe laser aperture (for the near and far radiation zones) is estimated taking into account the spatial coherence radius of the atmosphere r0, the radiation intensity and angular divergence of the laser beam, its random root-mean-square angular deviation (jitter) with respect to the calculated direction to the space objects. Main results. The estimation of the angular divergence of a laser beam is based on a comparative analysis and generalization of theoretical results obtained by calculating the optical resolution in systems for acquiring images of natural space objects. It is shown, in particular, that when determining the size of the aperture along with the value of the angular divergence of the probe beam, it is necessary to take into consideration the decrease in its radiation intensity with an increase in the diameter with respect to the radius of atmosphere coherence. The practical significance. The results are essential, firstly, for the development of ground-based adaptive optoelectronic systems for tracking artificial space objects, and secondly, for determining the geographic locations of the optoelectronic systems taking into account the astronomical climate.
Keywords: adaptive optical system, laser reference star, optical transfer function, atmospheric coherence radius, turbulent atmosphere, angular divergence of a laser beam, laser beam intensity
References
References
1. Lukin V.P. Adaptive optics in the formation of optical beams and images. Physics-Uspekhi, 2014, vol. 57, no. 6, pp. 556–592. doi: 10.3367/UFNe.0184.201406b.0599
2. Bolbasova L.A., Lukin V.P. Adaptive Correction of Atmospheric Distortions of optical Images Based on an Artificial Reference Source. Moscow, Fizmatlit Publ., 2012, 125 p. (in Russian)
3. Kleymyonov V.V., Novikova E.V. Existing large-sized ground optical telescopes for space objects observation. Journal of Instrument Engineering, 2018, vol. 61, no. 10, pp. 827–843. (in Russian).
4. Senft D., Hunt S., Swindle T.R., Morris N., Holmes R., Walker E., Lucas J., Toth J., Abercrombie M., Mooney J., Georges T. Sodium guidestar signal levels measured at AMOS and comparison to theory. Proc. of the Advanced Maui Optical and Space Surveillance Technologies Conference, 2019.
5. Bakut P.A., Ershova O.M., Shumilov Yu.P. Calculation of the energy of a synthetic laser beacon. Quantum Electronics, 1996, vol. 26, no. 12, pp. 1070–1074. doi: 10.1070/QE1996v026n12ABEH000876
6. Lukin V.P. Residual distortions caused by the size of a reference source. Atmospheric and Oceanic Optics, 2015, vol. 28, no. 2, pp. 107–114. doi: 10.1134/S1024856015020074
7. Lutomirski R.F., Yura H.T. Propagation of a finite optical beam in an inhomogeneous medium. Applied Optics, 1971, vol. 10, no. 7, pp. 1652–1658. doi: 10.1364/AO.10.001652
8. Ji X., Li X. Directionality of Gaussian array beams propagating in atmospheric turbulence. Journal of the Optical Society of America A: Optics and Image Science, and Vision, 2009, vol. 26, no. 2, pp. 236– 243.
9. Hardy J.W. Adaptive Optics for Astronomical Telescopes. Oxford University Press, 1998, 437 p.
10. Wang J. Optical resolution with adaptive phase compensation for light propagation in a turbulent atmosphere. Adaptive Optics, Moscow, Mir Publ., 1980, pp. 374–398. (in Russian)
11. Fried D.L. Optical resolution through a randomly inhomogeneous medium for very long and very short exposures. Journal of the Optical Society of America, 1966, vol. 56, no. 10, pp. 1372–1379. doi: 10.1364/JOSA.56.001372
12. Tatarskii V.I. Wave Propagation in a Turbulent Atmosphere. Moscow, Nauka Publ., 1967, 548 p. (in Russian)
13. Mironov V.L., Nosov V.V., Chen B.N. Quivering of optical images of laser sources in a turbulent atmosphere. Radiophysics and Quantum Electronics, 1980, vol. 23, no. 4, pp. 319–325. doi: 10.1007/BF01057640
14. Shanin O.I. Adaptive Optical Tilt Correction Systems. Resonant Adaptive Optics. Moscow, Technosphera Publ., 2013, 295 p. (in Russian)
15. Konyaev P.A., Kopulov E.A., Kovadlo P.G., Lukin V.P., Selin A.A., Shikhovtsev A.Yu. Works on a set of data measuring turbulence in different seasons of the year. Proceedings of SPIE, 2017, vol. 10466, pp. 104660N.
doi: 10.1117/12.2283000
16. Lukin V.P., Botygina N.N., Emaleev O.N., Lavrinov V.V. Peculiarities of adaptive phase correction of optical wave distortions under conditions of ‘strong’ intensity fluctuations. Quantum Electronics, 2020, vol. 50, no. 9, pp. 866–875. doi: 10.1070/QEL17302
17. Hulea M., Tang X., Ghassemlooy Z., Rajbhandari S. A review on effects of the atmospheric turbulence on laser beam propagation — An analytic approach. Proc 10th International Symposium on Communication Systems, Networks and Digital Signal Processing (CSNDSP), 2016, pp. 7573975. doi: 10.1109/CSNDSP.2016.7573975
18. Wang J.Y. Phase-compensated optical beam propagation through atmospheric turbulence. Applied Optics, 1978, vol. 17, no. 16, pp. 2580–2590. doi: 10.1364/AO.17.002580