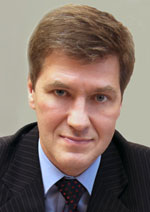
Nikiforov
Vladimir O.
D.Sc., Prof.
doi: 10.17586/2226-1494-2021-21-2-191-197
Numerical simulation of functional characteristics of solar elements InGaAsN/Si
Read the full article

For citation:
Devitsky O.V., Sanakulov S.O. Numerical simulation of functional characteristics of solar elements InGaAsN/Si. Scientific and Technical Journal of Information Technologies, Mechanics and Optics, 2021, vol. 21, no. 2, pp. 191–197 (in Russian). doi: 10.17586/2226-1494-2021-21-2-191-197
Abstract
For the first time, numerical simulation and optimization of the current-voltage characteristics of a fundamentally new single-junction solar cell based on the InxGa1–xAs1–yNy/Si heterostructure were carried out. The integration of multicomponent layers A3B5 and dilute nitrides A3B5N with silicon substrates is currently a technologically complex process. Despite the complexity of this integration, there are certain prerequisites that may make it possible to obtain InxGa1–xAs1–yNy layers with a relatively low defect density. The InxGa1–xAs1–yNy solid solution is quite promising for use in optoelectronics, but at the same time it is poorly studied. Numerical modeling was carried out using the AFORS HET v2.5 software product. When numerically calculating the parameters of the solar cell In0.02Ga0.98As1–yNy/Si, we obtained the following data: the nitrogen concentration y varied in the range from 0 to 5 %; the thickness of the In0.02Ga0.98As1–yNy layer varied within 0,3–0,8 μm; the degree of doping of the base and the emitter of the SC varied in the range 1016–8·1019 cm–3. The dependences of the current-voltage and spectral characteristics on the thickness and composition of the emitter, as well as the degree of doping of the layers were investigated. It is shown that solar cells consisting of the In0.02Ga0.98As0.98N0.02/Si heterojunction can achieve an efficiency of 22.2 % under illumination AM1.5. Modeling the effect of nitrogen concentration on the efficiency of solar cells showed that a change in nitrogen concentration from 0 to 5 % in the In0.02Ga0.98As1–yNy layer leads to a decrease in the efficiency of 21.9 % to 21.82 %, respectively. This fact is primarily due to a decrease in the value of the energy gap of the emitter and, as a consequence, to a decrease in the value of the open circuit voltage of the solar cell. It was found that an increase in the impurity concentration in the In0.02Ga0.98As0.98N0.02 emitter in the range 1016–8·1019 cm–3 leads to an increase in the solar cell efficiency from 17.11 % to 21.89 %, respectively. With an increase in the impurity concentration in the p-Si base in the range from 1016–5·1017 cm–3, a steady increase in efficiency is observed up to 22.2 %, and then a monotonic decrease to 10.87 % at an impurity concentration of 5·1017 cm–3. With a decrease in the emitter thickness, the quantum efficiency of a solar cell increases due to a decrease in the number of photogenerated charge carriers. As a result of numerical simulation in the AFORS HET v2.5 software product, it was determined that the open-circuit voltage of solar cells based on the n-In0.02Ga0.98As0.98N0.02/p-Si heterostructure is 716.8 mV, at a short-circuit current density of 36.52 mA/cm2, fill factor equal to 84.81 % and efficiency equal to 22.2 %.
Acknowledgements. The publication was prepared as a part of the state assignment “Development and creation of semiconductor heterointerfaces based on multicomponent materials for microwave electronics and photonics devices” for 2021 (state registration number AAAA-A19-119040390081-2).
References
- Shockley W., Queisser H.J. Detailed balance limit of efficiency of p-n junction solar cells. Journal of Applied Physics, 1961, vol. 32, no. 3, pp. 510–519. doi: 10.1063/1.1736034
- Bellil W., Aissat A., Vilcot J.P. Optimization and comparison between the efficiency of GaNAsSb and GaInNAs single solar cells deposed on GaAs. Procedia Computer Science, 2019, vol. 151, pp. 1028–1033. doi: 10.1016/j.procs.2019.04.145
- Blasco R., Naranjo F.B., Valdueza-Felip S. Design of AlInN on silicon heterojunctions grown by sputtering for solar devices. Current Applied Physics, 2020, vol. 20, no. 11, pp. 1244–1252. doi: 10.1016/j.cap.2020.07.018
- Essig S., Ward S., Steiner M.A., Friedman D.J., Geisz J.F., Stradins P., Young D.L. Progress towards a 30% efficient GaInP/Si tandem solar cell. Energy Procedia, 2015, vol. 77, pp. 464–469. doi: 10.1016/j.egypro.2015.07.066
- Kurtz S.R., Allerman A.A., Jones E.D., Gee J.M., Banas J.J., Hammons B.E. InGaAsN solar cells with 1.0 eV band gap, lattice matched to GaAs. Applied Physics Letters, 1999, vol. 74, no. 5, pp. 729–731. doi: 10.1063/1.123105
- Prete P., Lovergine N. Dilute nitride III-V nanowires for high-efficiency intermediate-band photovoltaic cells: Materials requirements, self-assembly methods and properties. Progress in Crystal Growth and Characterization of Materials, 2020, vol. 66, no. 4, pp. 100510. doi: 10.1016/j.pcrysgrow.2020.100510
- Kim T.W., Mawst L.J., Kim Y., Kim K., Lee J., Kuech T.F. 13.2% efficiency double-hetero structure single-junction InGaAsN solar cells grown by MOVPE. Journal of Vacuum Science & Technology A, 2015, vol. 33, no. 2, pp. 021205. doi: 10.1116/1.4906511
- Arbez G., Wheeldon J., Walker A., Hinzer K., Schriemer H. Modeling and simulation of triple junction solar cells. Proceedings of SPIE, 2010, vol. 7750, pp. 775032. doi: 10.1117/12.876131
- Cariou R., Benick J., Feldmann F., Höhn O., Hauser H., Beutel P., Razek N., Wimplinger M., Bläsi B., Lackner D., Hermle M., Siefer G., Glunz S.W., Bett A.W., Dimroth F. III–V-on-silicon solar cells reaching 33% photoconversion efficiency in two-terminal configuration. Nature Energy, 2018, vol. 3, no. 4, pp. 326–333. doi: 10.1038/s41560-018-0125-0
- Ganji J. Numerical simulation of thermal behavior and optimization of a-Si/a-Si/C-Si/a-Si/A-Si hit solar cell at high temperatures. Electrical Engineering & Electromechanics, 2017, no. 6, pp. 47–52. doi: 10.20998/2074-272X.2017.6.07
- Gudovskikh A.S., Kaluzhniy N.A., Lantratov V.M., Mintairov S.A., Shvarts M.Z., Andreev V.M. Numerical modelling of GaInP solar cells with AlInP and AlGaAs windows. Thin Solid Films, 2008, vol. 516, no. 20, pp. 6739–6743. doi: 10.1016/j.tsf.2007.12.016
- Aydin K., Leite M.S., Atwater H.A. Increased cell efficiency in InGaAs thin film solar cells with dielectric and metal back reflectors. Proc. 34th IEEE Photovoltaic Specialists Conference (PVSC), 2009, pp. 001713–001717. doi: 10.1109/PVSC.2009.5411432
- Lunin L.S., Lunina M.L., Devitsky O.V., Sysoev I.A. Pulsed laser deposition of AlxGa1–xAs and GaP thin films onto Si substrates for photoelectric converters. Semiconductors, 2017, vol. 51, no. 3, pp. 387–391. doi: 10.1134/S1063782617030174
- Varache R., Leendertz C., Gueunier-Farret M.E., Haschke J., Muñoz D., Korte L. Investigation of selective junctions using a newly developed tunnel current model for solar cell applications. Solar Energy Materials and Solar Cells, 2015, vol. 141, pp. 14–23. doi: 10.1016/j.solmat.2015.05.014
- Dilute III-V nitride semiconductors and material systems: Physics and Technology. Ed. by A. Erol. Berlin, Springer-Verlag, 2008, 590 p., Springer Materials Science, vol. 105. doi: 10.1007/978-3-540-74529-7
- Kudrawiec R. Alloying of GaNxAs1−x with InNxAs1−x: A simple formula for the band gap parametrization of Ga1−yInyNxAs1−x alloys. Journal of Applied Physics, 2007, vol. 101, no. 2, pp. 023522. doi: 10.1063/1.2424528
- Vurgaftman I., Meyer J.R., Ram-Mohan L.R. Band parameters for III-V compound semiconductors and their alloys. Journal of Applied Physics, 2001, vol. 89, no. 11, pp. 5815–5875. doi: 10.1063/1.1368156
- Vurgaftman I., Meyer J.R. Band parameters for nitrogen-containing semiconductors. Journal of Applied Physics, 2003, vol. 94, no. 6, pp. 3675–3696. doi: 10.1063/1.1600519
- Ajnef N., Jemmali W.Q., Habchi M.M., Rebey A. Biaxial strain effects on the band structure and absorption coefficient of GaAs1-x-yNxBiy/GaAs MQWs calculated using k.p method. Optik, 2020, vol. 223, pp. 165484. doi: 10.1016/j.ijleo.2020.165484
- Song J., Luo Z., Liu X., Li E., Jiang C., Huang Z., Li J., Guo X., Ding Z., Wang J. The study on structural and photoelectric properties of zincblende InGaN via first principles calculation. Crystals, 2020, vol. 10, no. 12, pp. 1159. doi: 10.3390/cryst10121159
- Kawamura T., Fujita Y., Hamaji Y., Akiyama T., Kangawa Y., Gorczyca I., Suski T., Wierzbowska M., Krukowski S. First‐principles calculation of band gaps of Al1−xInxN alloys and short period Al1−xInxN/Al1−yInyN superlattices. Physica Status Solidi B, 2020, vol. 257, no. 4, pp. 1900530. doi: 10.1002/pssb.201900530
- Geppert T., Wagner J., Köhler K., Ganser P., Maier M. Preferential formation of Al–N bonds in low N-content AlGaAsN. Applied Physics Letters, 2002, vol. 80, no. 12, pp. 2081–2083. doi: 10.1063/1.1464660