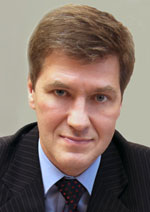
Nikiforov
Vladimir O.
D.Sc., Prof.
doi: 10.17586/2226-1494-2021-21-2-283-296
The influence of viscosity and turbulence on the supersonic flow compression and expansion corner
Read the full article

For citation:
Volkov K.N., Ilina E.E., Volobuev I.A. The influence of viscosity and turbulence on the supersonic flow compression and expansion corner. Scientific and Technical Journal of Information Technologies, Mechanics and Optics, 2021, vol. 21, no. 2, pp. 283–296 (in Russian). doi: 10.17586/2226-1494-2021-21-2-283-296
Abstract
Super- and hypersonic flow around aircraft elements is accompanied by the formation of a complex flow structure, which is characterized by the presence of strong shock waves, rarefaction waves, contact discontinuities, separation and reattachment of the flow. For such problems, the interaction of shock waves with viscous boundary layers is characteristic. Such interaction is quite complex and largely determines the effectiveness of aircraft. When flowing around complex structures of aircraft and their power plants in the vicinity of convex corner points of the geometry, compression and expansion flows are locally realized. The calculation of oblique shock waves, formed when flowing around the compression angle, and simple expansion waves, formed around the flowing angle of expansion, is included as an element of solving many problems of constructing streamlines and finding the pressure distribution on the streamlined surface. Based on the technology of adaptive meshes, a numerical model is proposed for studying two-dimensional effects arising from supersonic flow around the angles of compression and expansion. Numerical simulation was performed using various models such as inviscid, laminar or turbulent. In the calculations, the Mach number of the incident shock wave varies from 2 to 15, and the flow angle varies from 5 to 15 degrees. The Reynolds number, calculated from the characteristics of the unperturbed flow, is equal to 105. The working gas is air (γ = 1.4). A qualitative picture of the flow during diffraction of a shock wave on steps of different geometry is considered. When the shock wave interacts with the compression angle, flow separation and the formation of a recirculation region are observed, and when the shock wave interacts with the expansion angle, the Prandtl–Mayer wave is misaligned. The results of calculations are compared with theoretical data on the parameters of the flow behind the shock front or fan of the rarefaction wave. Studies have shown the influence of the effects of viscosity and turbulence on the flow structure and distribution of flow characteristics when flowing around the angles of compression and expansion. The simulation results can find application in solving problems related to the design of air intakes of high-speed flying aircrafts, in particular, in parametric and optimization calculations of gas-dynamic flows that arise in the elements of propulsion systems of supersonic and hypersonic aircraft.
Acknowledgements. This work was financially supported by the Ministry of Science and Higher Education of Russian Federation during the implementation of the project “Creating a leading scientific and technical reserve in the development of advanced technologies for small gas turbine, rocket and combined engines of ultra-light launch vehicles, small spacecraft and unmanned aerial vehicles that provide priority positions for Russian companies in emerging global markets of the future”, No. FZWF-2020-0015.
References
- Anderson J.D. Modern Compressible Flow: With Historical Perspective. McGraw-Hill, 1990, 671 с.
- Dolling D. Fifty years of shock-wave/boundary-layer interaction research: what next? AIAA Journal, 2001, vol. 39, no. 8, pp. 1517–1531. doi: 10.2514/2.1476
- Flower J.W. Configurations for high supersonic speeds derived from simple shock-waves and expansions. The Aeronautical Journal, 1963, vol. 67, no. 629, pp. 287–290. doi: 10.1017/S0368393100078597
- Bourdon C.J., Dutton J.C. Effects of boattailing on the turbulence structure of a compressible base flow. Journal of Spacecraft and Rockets, 2001, vol. 38, no. 4, pp. 534–541. doi: 10.2514/2.3736
- Knight D., Yan H., Panaras A.G., Zheltovodov A. Advances in CFD prediction of shock wave turbulent boundary layer interactions. Progress in Aerospace Sciences, 2003, vol. 39, no. 2-3, pp. 121–184. doi: 10.1016/S0376-0421(02)00069-6
- Roy C.J., Blottner F.G. Review and assessment of turbulence models for hypersonic flows. Progress in Aerospace Sciences, 2006, vol. 42, no. 7-8, pp. 469–530. doi: 10.1016/j.paerosci.2006.12.002
- Ghia U., Bayyuk S., Habchi S., Roy C., Shih T., Conlisk T., Hirsch C., Powers J.M. The AIAA code verification project - test cases for CFD code verification. Proc. 48th AIAA Aerospace Sciences Meeting Including the New Horizons Forum and Aerospace Exposition, 2010, pp. 2010-0125. doi: 10.2514/6.2010-125
- Araya G. Turbulence model assessment in compressible flows around complex geometries with unstructured grids. Fluids, 2019, vol. 4, no. 2, pp. 4020081. doi: 10.3390/fluids4020081
- Settles G.S., Fitzpatrick T.J., Bogdonoff S.M. Detailed study of attached and separated compression corner flowfields in high Reynolds number supersonic flow. AIAA Journal, 1979, vol. 17, no. 6, pp. 579–585. doi: 10.2514/3.61180
- Smits A.J, Muck K.-C. Experimental study of three shock wave/turbulent boundary layer interactions. Journal of Fluid Mechanics, 1987, vol. 182, pp. 291–314. doi: 10.1017/S0022112087002349
- Kuntz D.W., Amatucci V.A., Addy A.L. Turbulent boundary-layer properties downstream of the shock-wave/boundary-layer interaction. AIAA Journal, 1987, vol. 25, no. 5, pp. 668–675. doi: 10.2514/3.9681
- Evans T.T., Smits A.J. Measurements of the mean heat transfer in a shock wave-turbulent boundary layer interaction. Experimental Thermal and Fluid Science, 1996, vol. 12, no. 1, pp. 87–97. doi: 10.1016/0894-1777(95)00078-X
- Nguyen T., Behr M., Reinartz U., Hohn O., Gülhan A. Numerical investigations of the effects of sidewall compression and relaminarization in 3D scramjet inlet. Proc. 17th AIAA International Space Planes and Hypersonic Systems and Technologies Conference, 2011, pp. 2011–2256. doi: 10.2514/6.2011-2256
- Chuvakhov P.V. A Riemann solver for RANS. Computational Mathematics and Mathematical Physics, 2014, vol. 54, no. 1, pp. 135–147. doi: 10.1134/S0965542514010072
- Fang J., Yao Y., Zheltovodov A., Li Z., Lu L. Direct numerical simulation of supersonic turbulent flows around a tandem expansion-compression corner. Physics of Fluids, 2015, vol. 27, no. 12, pp. 125104. doi: 10.1063/1.4936576
- Pasha A.A., Juhany K.A. Numerical simulation of compression corner flows at Mach number 9. Chinese Journal of Aeronautics, 2020, vol. 33, no. 6, pp. 1611–1624. doi: 10.1016/j.cja.2020.01.005
- Gai S.L., Khraibut A. Hypersonic compression corner flow with large separated regions. Journal of Fluid Mechanics, 2019, vol. 877, pp. 471–494. doi: 10.1017/jfm.2019.599
- Neiland V.Ia., Sokolov L.A., Shvedchenko V.V. Separation flow structure in supersonic flow past a compression corner at different values of the temperature factor. Advances in Continuum Mechanics. On the 70th Anniversary of Academician V.A. Levin, Vladivostok, 2009, pp. 540–562. (in Russian)
- Shvedchenko V.V. 3D secondary separation at supersonic flow over the compression RAMP. TsAGI Science Journal, 2010, vol. 41, no. 6, pp. 631–650. doi: 10.1615/TsAGISciJ.v41.i6.30
- Doǧrusöz S., Kavsaoǧlu M.Ş., Kaynak Ü. Numerical solution of hypersonic compression corner flows. Proc. 10th AIAA/NAL-NASDA-ISAS International Space Planes and Hypersonic Systems and Technologies Conference, 2001, pp. 2001-1750. doi: 10.2514/6.2001-1750
- Edwards J.R., Choi J.-I., Boles J.A. Large-eddy/Reynolds-averaged Navier-Stokes simulation of a Mach 5 compression-corner interaction. AIAA Journal, 2008, vol. 46, no. 4, pp. 977–991. doi: 10.2514/1.32240
- Gieseking D., Edwards J., Choi J. Simulation of a Mach 3 24-degree compression-ramp interaction using LES/RANS models. Proc. 47th AIAA/ASME/SAE/ASEE Joint Propulsion Conference and Exhibit, 2011, pp. 2011–5541. doi: 10.2514/6.2011-5541
- Charwat A.F., Yakura J.K. An investigation of two-dimensional supersonic base pressure. Journal of the Aerospace Sciences, 1958, vol. 25, no. 2, pp. 122–128. doi: 10.2514/8.7526
- Scherberg M.G., Smith H.E. An experimental study of supersonic flow over a rearward facing step. AIAA Journal, 1967, vol. 5, no. 1, pp. 51–56. doi: 10.2514/3.3906
- Bedarev I.A., Fedorova N.N. Structure of supersonic turbulent flows in the vicinity of inclined backward-facing steps. Journal of Applied Mechanics and Technical Physics, 2006, vol. 47, no. 6, pp. 812–820. doi: 10.1007/s10808-006-0119-8
- Chung K.-M., Lu F.K. Hypersonic turbulent expansion-corner flow with shock impingement. Journal of Propulsion and Power, 1995, vol. 11, no. 3, pp. 441–447. doi: 10.2514/3.23863
- Arnette S., Samimy M., Elliott G. Structure of supersonic turbulent boundary layer after expansion regions. AIAA Journal, 1995, vol. 33, no. 3, pp. 430–438. doi: 10.2514/3.60007
- Arnette S., Samimy M., Elliott G. The effects of expansion on the turbulence structure of compressible boundary layers. Journal of Fluid Mechanics, 1998, vol. 367, pp. 67–105. doi: 10.1017/S0022112098001475
- Tong F., Chen J., Sun D., Li X. Wall-shear stress fluctuations in a supersonic turbulent boundary layer over an expansion corner. Journal of Turbulence, 2020, vol. 21, no. 7, pp. 355–374. doi: 10.1080/14685248.2020.1797058
- Schlatter P., Örlü R. Assessment of direct numerical simulation data of turbulent boundary layers. Journal of Fluid Mechanics, 2010, vol. 659, pp. 116–126. doi: 10.1017/S0022112010003113
- Sun M.-B., Hu Z., Sandham N.D. Recovery of a supersonic turbulent boundary layer after an expansion corner. Physics of Fluids, 2017, vol. 29, no. 7, pp. 076103. doi: 10.1063/1.4995293
- Surov V.S. The Godunov method for calculating multidimensional flows of a one-velocity multicomponent mixture. Journal of Engineering Physics and Thermophysics, 2016, vol. 89, no. 5, pp. 1227–1240. doi: 10.1007/s10891-016-1486-5
- Volkov K. Multigrid and preconditioning techniques in CFD applications. CFD Techniques and Thermo-Mechanics Applications. Springer International Publishing, 2018, pp. 83–149. doi: 10.1007/978-3-319-70945-1_6
- Struchkov A.V., Kozelkov A.S., Volkov K.N., Kurkin A.A., Zhuckov R.N., Sarazov A.V. Numerical simulation of aerodynamic problems based on adaptive mesh refinement method. Acta Astronautica, 2020, vol. 172, pp. 7–15. doi: 10.1016/j.actaastro.2020.03.019