Menu
Publications
2024
2023
2022
2021
2020
2019
2018
2017
2016
2015
2014
2013
2012
2011
2010
2009
2008
2007
2006
2005
2004
2003
2002
2001
Editor-in-Chief
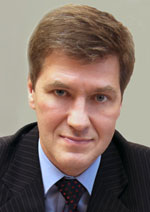
Nikiforov
Vladimir O.
D.Sc., Prof.
Partners
doi: 10.17586/2226-1494-2022-22-1-138-146
A study of the efficiency of the magnetic compass correction system
Read the full article

Article in Russian
For citation:
Abstract
For citation:
Gryazin D.G., Paderina T.V. A study of the efficiency of the magnetic compass correction system. Scientific and Technical Journal of Information Technologies, Mechanics and Optics, 2022, vol. 22, no. 1, pp. 138–146 (in Russian). doi: 10.17586/2226-1494-2022-22-1-138-146
Abstract
In sailing conditions under the rolling, dynamic compass errors may appear due to the influence of the redistributed magnetic masses of the ship, as well as of centripetal and tangential accelerations. The influence of these errors can be compensated by introducing a correction system into the measuring circuit of the compass, which uses one gyroscopic angular rate sensor. The correction method is based on the use of a gyroscopic angular rate sensor with a vertical axis of sensitivity in the circuit. The generated signal is the difference between the output reading of the magnetic compass and the integrated signal of the angular rate sensor, which is insensitive to the action of translational acceleration and redistributed magnetic masses during the ship’s heel. The resulting difference will contain the compass error from the rolling effect, further compensated for in the compass output signal. The choice of the parameters of the dynamic links of the circuit for the implementation of the correction system and the study of its operation was carried out by simulation method using the proposed analytical expression for the error of the magnetic compass from the rolling effect. Comparison of the simulation results with the results of experimental studies of the compass was carried out using a specialized stand that simulates the yaw of a ship and allows one to change the value of the vector of the Earth’s magnetic field, when exposed to the magnetic system of the compass. Experimental studies of the compass showed that the pitching error correction coefficient is in the range 0.16-0.48 (average value is 0.35), when the object’s angular oscillation periods change from 6 to 28 s, which characterizes the degree of pitching suppression, and when modeling, the average value of the coefficient is 0.21. The underestimation of the correction factor in modeling is due to the lack of taking into account the dynamic properties of the compass rose and depends on the ratio of the periods of natural oscillations of the compass and the periods of disturbing influences. The results confirmed the high efficiency of the considered compass correction system and the required quality of the developed specialized stand for evaluating its work. The results of the study can be used in the development of modern magnetic compasses to ensure high accuracy of directional guidance through the use of the proposed correction system.
Keywords: magnetic compass, ship pitching, compass correction system, gyroscopic angular rate sensor
Acknowledgements. This work was supported by the RFBR grant No. 20-08-00265. The authors express their gratitude to the employees of State Research Center of the Russian Federation Concern CSRI Elektropribor, JSC, K.S. Gorokhovsky and I.V. Sergachev, who participated in the development of the yaw stand and the study of the characteristics of the magnetic compass correction system on this stand.
References
Acknowledgements. This work was supported by the RFBR grant No. 20-08-00265. The authors express their gratitude to the employees of State Research Center of the Russian Federation Concern CSRI Elektropribor, JSC, K.S. Gorokhovsky and I.V. Sergachev, who participated in the development of the yaw stand and the study of the characteristics of the magnetic compass correction system on this stand.
References
-
Kardashinskii-Braude L.A. Deviations of Magnetic Compasses. Leningrad, CNII Rumb, 1986, 81 p. (in Russian)
-
Voronov V.V., Kardashinskii-Braude L.A. Universal latitude compensator for magnetic compass deviation. NavigationandGyroscopy, 1990, no. 2. (in Russian)
-
Arribalzaga J., Martinez A.T., Vila J.A. Analysis and calculation of the magnetic moment of a magnet compensation for type A magnetic needle. Journal of Maritime Research, 2013, vol. 10, no. 2, pp. 80–81.
-
Androjna A., Belev B., Pavic I., Perkovič M. Determining residual deviation and analysis of the current use of the magnetic compass. Journal of Marine Science and Engineering, 2021, vol. 9, no. 2, pp. 1–15. https://doi.org/10.3390/jmse9020204
-
Van Suong Nguyen. Calculation of the deviation coefficients for marine magnetic compass. Journal of International Maritime Safety, Environmental Affairs and Shipping, 2019, vol. 2, no. 2, pp. 112–115. https://doi.org/10.1080/25725084.2019.1569336
-
Meleshko V.V., Lakoza S.L., Sharov S.A. Method of identifying and eliminating magnetic compass deviation. Proc. of the IEEE 1st Ukraine Conference on Electrical and Computer Engineering (UKRCON 2017), 2017, pp. 288–291. https://doi.org/10.1109/UKRCON.2017.8100494
-
Iribar I.B., Munoz J.A.V., Labajos C.A.P. Latitude error in compass deviation: Mathematical method to determine the latitude error in magnetic compass deviation. Polish Maritime Research, 2014, vol. 21, no. 3, pp. 25–31. https://doi.org/10.2478/pomr-2014-0026
-
Rybaltovskii N.Iu. Magnetic Compass. Leningrad, Vodtransizdat Publ., 1954, 492 c. (in Russian)
-
Gryazin D.G., Sergachev I.V. Influence of external factors on transient processes of magnetic compass at high latitudes. Journal of Instrument Engineering, 2020, vol. 63, no. 10, pp. 921–929. (in Russian). https://doi.org/10.17586/0021-3454-2020-63-10-921-929
-
Gryazin D.G., Paderina T.V., Sergachev I.V. On the possibility of using ship's magnetic compasses at high latitudes. Proc. of the 32nd conference in memory of N.N. Ostryakov, St. Petersburg, Concern CSRI Elektropribor, 2020, pp. 122–125. (in Russian)
-
Gryazin D.G., Paderina T.V. Mechatronic control devices for a modern ship’s magnetic compass. Mekhatronika, Avtomatizatsiya, Upravlenie, 2021, vol. 22, no. 8, pp. 425–432. (in Russian). https://doi.org/10.17587/mau.22.425-432
-
Lushnikov E. Magnetic compass in modern maritime navigation. TransNav. International Journal on Marine Navigation and Safety of Sea Transportation, 2015, vol. 9, no. 4, pp. 539–543. https://doi.org/10.12716/1001.09.04.10
-
Lushnikov E. The reliability of compass information at navigational safety. Scientific Journals of the Maritime University of Szczecin, 2012, no. 29, pp. 117–121.
-
Basterretxea-Iribal I., Sotés I., Uriarte J.I. Towards an improvement of magnetic compass accuracy and adjustment. Journal of Navigation, 2016, vol. 69, no. 6, pp. 1325–1340. https://doi.org/10.1017/S0373463316000138
-
Kazakova G.F., Kardashinskii-Braude L.A., Fomkin Ia.I. Ship Induction and Hydromagnetic Compasses, Needle Magnetic Compasses with Inductive Sensors. Leningrad, NPO “Azimut”, 1991, 62 с. (in Russian)
-
Kardashinskii-Braude L.A. Modern Marine Magnetic Compasses. St. Petersburg, Concern CSRI Elektropribor, 1999, 137 p. (in Russian)
-
Nechaev P.A., Grigorev V.V. Magnetic Compass. Moscow, Transport Publ., 1983, 240 p. (in Russian)
-
Kardashinskii-Braude L.A. Increasing the dynamic stability of ship's magnetic compass readings at horizontal accelerations. Available at: ttp://www.navydevices.ru/informations/section-publications/publications/85-2002-09-21-13-29-52 (accessed: 23.07.2020). (in Russian)
-
Krylov A.N. On perturbations of compass readings that occur on the rolling of a ship in waves. Izbrannye trudy akademika A.N. Krylova. Moscow, Academy of Sciences of the Soviet Union, 1958, pp. 115–170. (in Russian)
-
Zinenko V.M., Gryazin D.G., Molochnikov A.A., Sergachev I.V., Matveev Y.V., Korolenko I.V. Method for measuring ship magnetic course in high latitudes and device for its implementation. Patent RU2688900C1, 2019. (in Russian)
-
Boronachin A.M., Lukomski Y.A., Shpektorov A.G., Ha Manh Thang, Tran Tang Duc. Yaw-and-vertical gyro unit for high-speed marine vehicle motion control. Proceedings of Saint Petersburg Electrotechnical University, 2012, no. 4, pp. 75–80. (in Russian)
-
Boronachin A.M., Lukomski Y.A., Shpektorov A.G., Ha Manh Thang, Tran Tang Duc. The basis of navigation system requirements for solving a problem of marine vehicles motion control. Proceedings of Saint Petersburg Electrotechnical University, 2012, no. 2, pp. 77–80. (in Russian)
-
Kozhukhov V.P., Voronov V.V., Grigorev V.V. Magnetic Compasses. Moscow, Transport Publ., 1981, 212 p. (in Russian)
-
Borodai I.K., Morenshildt V.A., Vilenskii G.V. Applied Problems of Ship Dynamics in Waves. Leningrad, Sudostroenie Publ., 1989, 258 p. (in Russian)