Menu
Publications
2025
2024
2023
2022
2021
2020
2019
2018
2017
2016
2015
2014
2013
2012
2011
2010
2009
2008
2007
2006
2005
2004
2003
2002
2001
Editor-in-Chief
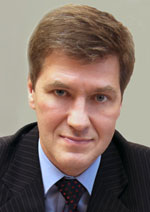
Nikiforov
Vladimir O.
D.Sc., Prof.
Partners
doi: 10.17586/2226-1494-2022-22-2-392-400
Throughput modeling of cellular network systems with spatial precoding
Read the full article

Article in Russian
For citation:
Abstract
For citation:
Medvedev A.S., Ivanov V.V. Throughput modeling of cellular network systems with spatial precoding. Scientific and Technical Journal of Information Technologies, Mechanics and Optics, 2022, vol. 22, no. 2, pp. 392–400 (in Russian). doi: 10.17586/2226-1494-2022-22-2-392-400
Abstract
The paper discusses wireless cellular radio communication systems along highways using Multiple-input Multiple-output (MIMO, multiple transmit / receive antennas) technologies, in particular spatial multiplexing and diversity reception, and also proposes a model for assessing the potential gains in a multi-antenna system from MIMO, which takes into account the multipath of the channel and the relative orientation of the antennas. The work was carried out by transferring the results of the calculated correlation matrix from stochastic channel model into the physical layer simulator of the cellular system protocol. A methodology for evaluating the performance of multi-antenna systems using spatial multiplexing for roadside cellular networks has been developed. The correlation properties of the channel between the antennas of the two roadside units (RSU), each of which has two perpendicular linearly polarized antennas and a user terminal with the same two orthogonally polarized antennas, have been investigated. A prediction scheme for the type of correlation matrix has been developed, which makes it possible to more accurately set the correlation matrix in simulators of the physical layer. The obtained results showed that for properly designed systems the throughput will be close to the throughput of low spatial correlation, and the case of high correlation proposed by the standard does not need to be modeled. It is also shown that the channels between Tx / Rx pairs that undergo similar polarization changes (the same relative spatial rotation of the antennas) will be strongly correlated, which must be taken into account when developing MIMO systems.
Keywords: MIMO, polarization, radio channel, spatial multiplexing, diversity reception
References
References
-
Kryukov Y.V., Pokamestov D.A., Rogozhnikov E.V., Novichkov S.A., Lakontsev D.V. Analysis of computational complexity and processing time evaluation of the protocol stack in 5G new radio. Proceedings of TUSUR University, 2020, vol. 23, no. 3, pp. 31–37. (in Russian). https://doi.org/10.21293/1818-0442-2020-23-3-31-37
-
Sergeev A.M., Blaunstein N.Sh. Evolution of multiple-access networks – cellular and non-cellular – in historical perspective. Part 4. Informatsionno-upravliaiushchie sistemy, 2019, no. 1, pp. 65–75. https://doi.org/10.31799/1684-88532019-1-65-75
-
Starovoytov M.Yu. The study of performance limits of receive antenna selection for MIMO spatial multiplexing in non-stationary channel. T-Comm, 2017, vol. 11, no. 8, pp. 63–68.
-
ICT-317669-METIS/D8.4 Project Name: Mobile and wireless communications Enablers for the Twenty-twenty Information Society (METIS). Deliverable D8.4 METIS final project report, Date of delivery: 30.04.2015.
-
Weiler R.J., Peter M., Keusgen W., Maltsev A., Karls I., Pudeyev A., Bolotin I., Siaud I., Ulmer-Moll A.-M. Quasi-deterministic millimeter-wave channel models in MiWEBA. EURASIP Journal on Wireless Communications and Networking, 2016, no. 1, pp. 84. https://doi.org/10.1186/s13638-016-0568-6
-
Kanhere O., Rappaport T.S. Position Location for futuristic cellular communications: 5G and beyond. IEEE Communications Magazine, 2021, vol. 59, no. 1, pp. 70–75. https://doi.org/10.1109/MCOM.001.2000150
-
Liu L., Oestges C., Poutanen J., Haneda K., Vainikainen P., Quitin F., Tufvesson F., Doncker P. The COST 2100 MIMO channel model. IEEE Wireless Communications, 2012, vol. 19, no. 6, pp. 92–99. https://doi.org/10.1109/MWC.2012.6393523
-
Busche H., Vanaev A., Rohling H. SVD-based MIMO precoding and equalization schemes for realistic channel knowledge: Design criteria and performance evaluation. Wireless Personal Communications, 2009, vol. 48, no. 3, pp. 347–359. https://doi.org/10.1007/s11277-008-9526-x
-
3GPP TR 38.901. 5G; Study on channel model for frequencies from 0.5 to 100 GHz. v16.1.0.Jan. 2020. Available at: https://www.etsi.org/deliver/etsi_tr/138900_138999/138901/16.01.00_60/tr_138901v160100p.pdf (accessed: 01.03.2022).
-
Jaeckel S., Raschkowski L., Thiele L., Burkhardt F., Eberlein E. QuuaDRiGa: Quasi Deterministic Radio Channel Generator, User Manual and Documentation. Document Revision v2.0.0. 2017. Fraunhofer Heinrich Hertz Institute, 2017.
-
3GPP TR 36.101. Evolved Universal Terrestrial Radio Access (E-UTRA); User Equipment (UE) radio transmission and reception. V10.3.0 (2011-06). Available at: https://www.etsi.org/deliver/etsi_ts/136100_136199/136101/10.03.00_60/ts_136101v100300p.pdf (acessed: 01.03.2022).
-
3GPP TS 38.211 5G; NR; Physical channels and modulation. V15.2.0 (2018-07). Available at: https://www.etsi.org/deliver/etsi_ts/138200_138299/138211/15.02.00_60/ts_138211v150200p.pdf (accessed).
-
3GPP TS 38.214 5G; NR; Physical layer procedures for data V15.3.0 (2018-10). Available at: https://www.etsi.org/deliver/etsi_ts/138200_138299/138214/15.03.00_60/ts_138214v150300p.pdf (accessed: 01.03.2022).