Menu
Publications
2025
2024
2023
2022
2021
2020
2019
2018
2017
2016
2015
2014
2013
2012
2011
2010
2009
2008
2007
2006
2005
2004
2003
2002
2001
Editor-in-Chief
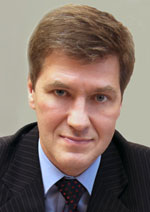
Nikiforov
Vladimir O.
D.Sc., Prof.
Partners
doi: 10.17586/2226-1494-2022-22-3-509-516
Photocatalytic properties of Ag-AgBr nanostructures in ion-exchanged layers of bromide sodium-zinc-aluminosilicate glass matrix
Read the full article

Article in English
For citation:
Abstract
For citation:
Marasanov D.V., Sgibnev Y.M., Nikonorov N.V. Photocatalytic properties of Ag–AgBr nanostructures in ion-exchanged layers of bromide sodium-zinc-aluminosilicate glass matrix. Scientific and Technical Journal of Information Technologies, Mechanics and Optics, 2022, vol. 22, no. 3, pp. 509–516. (in Russian). doi: 10.17586/2226-1494-2022-22-3-509-516
Abstract
The photocatalytic properties of Ag–AgBr nanostructures formed by low-temperature ion exchange method and followed heat-treatment in bromide sodium-zinc-aluminosilicate glass have been investigated. Glasses based on Na2O–ZnO–Al2O3–SiO2 system and doped with Sb2O3, Ce2О3 and Br were synthesized. Layers containing silver ions were formed on the surface of sodium-zinc-aluminosilicate glass by the ion exchange method. The glass samples were immersed in a bath containing a melt of nitrate mixture 5AgNO3/95NaNO3 (mol%) at 320 °C for 2 hours. Subsequent heat treatment at 500 °C resulted in a formation of Ag–AgBr nanostructures in the surface layer. The photocatalytic properties of the Ag–AgBr nanostructures on the glass surface were measured by the decomposition of the methyl orange dye. A comprehensive study of the spectral and photocatalytic properties of Ag–AgBr nanostructures has been carried out. It was shown that, after ion exchange and heat treatment, the AgBr crystal shells with a size of 6 nm were formed around silver nanoparticles. It has been established that the presence of a photocatalyst with Ag–AgBr nanostructures in the surface layer of glass under ultraviolet irradiation leads to degradation of the methyl orange dye by 77 %. Reducing the thickness of the ion-exchange layer to 5 μm by chemical etching decreased the degradation efficiency of the methyl orange dye to 15 %. The results of the work can be applied in devices for the photocatalytic decomposition of water to produce hydrogen.
Keywords: ion exchange, bromide sodium-zinc-aluminosilicate glass, silver nanoparticles, AgBr nanocrystals, photocatalysis, methyl orange dye
Acknowledgements. The work was supported by the Russian Science Foundation (project no. 20-19-00559). The authors thank engineer Kuzmenko N.K. in ITMO University for X-ray phase analysis.
References
Acknowledgements. The work was supported by the Russian Science Foundation (project no. 20-19-00559). The authors thank engineer Kuzmenko N.K. in ITMO University for X-ray phase analysis.
References
-
Sosna M.Kh., Kryuchkov M.V., Maslennikova M.V., Pustovalov M.V. "Green" and/or "Blue" hydrogen. Oil & Gas Chemistry, 2020, no. 3-4, pp. 21–23. (in Russian). https://doi.org/10.24412/2310-8266-2020-3-4-21-23
-
Fu X., Chang H., Shang Z., Liu P., Liu J., Luo H. Three-dimensional Cu2O nanorods modified by hydrogen treated Ti3C2TX MXene with enriched oxygen vacancies as a photocathode and a tandem cell for unassisted solar water splitting. Chemical Engineering Journal, 2020, vol. 381, pp. 122001. https://doi.org/10.1016/j.cej.2019.122001
-
Wang L., Si W., Hou X., Wang M., Liu X., Ye Y., Hou F., Liang J. Novel integrated strategies toward efficient and stable unassisted photoelectrochemical water splitting. Sustainable Materials and Technologies, 2020, vol. 25, pp. e00209. https://doi.org/10.1016/j.susmat.2020.e00209
-
Liu Z., Hou W., Pavaskar P., Aykol M., Cronin S.B. Plasmon resonant enhancement of photocatalytic water splitting under visible illumination. Nano Letters, 2011, vol. 11, no. 3, pp. 1111–1116. https://doi.org/10.1021/nl104005n
-
Hu Q., Liu B., Zhang Z., Song M., Zhao X. Temperature effect on the photocatalytic degradation of methyl orange under UV-vis light irradiation. Journal of Wuhan University of Technology-Mater Science Edition, 2010, vol. 25, no. 2, pp. 210–213. https://doi.org/10.1007/s11595-010-2210-5
-
Kumar P., Govindaraju M., Senthamilselvi S., Premkumar K. Photocatalytic degradation of methyl orange dye using silver (Ag) nanoparticles synthesized from Ulva lactuca. Colloids and Surfaces B: Biointerfaces, 2013, vol. 103, pp. 658–661. https://doi.org/10.1016/j.colsurfb.2012.11.022
-
Jiang J., Li H., Zhang L. New insight into daylight photocatalysis of AgBr@Ag: Synergistic effect between semiconductor photocatalysis and plasmonic photocatalysis. Chemistry - A European Journal, 2012, vol. 18, no. 20, pp. 6360–6369. https://doi.org/10.1002/chem.201102606
-
Wang H., Gao J., Guo T., Wang R., Guo L., Liu Y., Li J. Facile synthesis of AgBr nanoplates with exposed {111} facets and enhanced photocatalytic properties. Chemical Communications, 2012, vol. 48, no. 2, pp. 275–277. https://doi.org/10.1039/c1cc16423f
-
Dong R., Tian B., Zeng C., Li T., Wang T., Zhang J. Ecofriendly synthesis and photocatalytic activity of uniform cubic Ag@AgCl plasmonic photocatalyst. Journal of Physical Chemistry C, 2013, vol. 117, no. 1, pp. 213–220. https://doi.org/10.1021/jp311970k
-
Han L., Wang P., Zhu C., Zhai Y., Dong S. Facile solvothermal synthesis of cube-like Ag@AgCl: a highly efficient visible light photocatalyst. Nanoscale, 2011, vol. 3, no. 7, pp. 2931–2935. https://doi.org/10.1039/c1nr10247h
-
Wang P., Huang B., Zhang X., Qin X., Jin H., Dai Y., Wang Z., Wei J., Zhan J., Wang S., Wang J., Whangbo M. Highly efficient visible-light plasmonic photocatalyst Ag@AgBr. Chemistry - A European Journal, 2009, vol. 15, no. 8, pp. 1821–1824. https://doi.org/10.1002/chem.200802327
-
Saravanan R., Manoj D., Qin J., Naushad M., Gracia F., Lee A.F., Khan M.M., Gracia-Pinilla M.A. Mechanothermal synthesis of Ag/TiO2 for photocatalytic methyl orange degradation and hydrogen production. Process Safety and Environmental Protection, 2018, vol. 120, pp. 339–347. https://doi.org/10.1016/j.psep.2018.09.015
-
Shakeel M., Li B., Arif M., Yasin G., Rehman W., Khan A.U., Khan S., Khan A., Ali J. Controlled Synthesis of highly proficient and durable hollow hierarchical heterostructured (Ag-AgBr/HHST): A UV and Visible light active photocatalyst in degradation of organic pollutants. Applied Catalysis B: Environmental, 2018, vol. 227, pp. 433–445. https://doi.org/10.1016/j.apcatb.2018.01.037
-
Li L., Yang X., Xie C., Wang Y., Wei L., Yang J. Synthesis and photocatalytic mechanism of visible-light-driven Ag/AgBr/(I/S) composites for organic dyes degradation. Optical Materials, 2022, vol. 123, pp. 111947. https://doi.org/10.1016/j.optmat.2021.111947
-
Sgibnev Y.M., Nikonorov N.V., Ignatiev A.I. High efficient luminescence of silver clusters in ion-exchanged antimony-doped photo-thermo-refractive glasses: Influence of antimony content and heat treatment parameters. Journal of Luminescence, 2017, vol. 188, pp. 172–179. https://doi.org/10.1016/j.jlumin.2017.04.028
-
Sgibnev Y., Asamoah B., Nikonorov N., Honkanen S. Tunable photoluminescence of silver molecular clusters formed in Na+-Ag+ ion-exchanged antimony-doped photo-thermo-refractive glass matrix. Journal of Luminescence, 2020, vol. 226, pp. 117411. https://doi.org/10.1016/j.jlumin.2020.117411
-
Dubrovin V., Nikonorov N., Ignatiev A. Bromide photo-thermo-refractive glass for volume Bragg gratings and waveguide structure recording. Optical Materials Express, 2017, vol. 7, no. 7, pp. 2280–2292. https://doi.org/10.1364/OME.7.002280
-
Dubrovin V.D., Ignatiev A.I., Nikonorov N.V. Chloride photo-thermo-refractive glasses. Optical Materials Express, 2016, vol. 6, no. 5, pp. 1701–1713. https://doi.org/10.1364/ome.6.001701
-
Sgibnev Y., Nikonorov N., Ignatiev A. Governing functionality of silver ion-exchanged photo-thermo-refractive glass matrix by small additives. Applied Sciences, 2021, vol. 11, no. 9, pp. 3891. https://doi.org/10.3390/app11093891
-
White J.M., Heidrich P.F. Optical waveguide refractive index profiles determined from measurement of mode indices: a simple analysis. Applied Optics, 1976, vol. 15, no. 1, pp. 151–155. https://doi.org/10.1364/AO.15.000151
-
Patterson A.L. The Scherrer formula for X-ray particle size determination. Physical Review, 1939, vol. 56, no. 10, pp. 978.https://doi.org/10.1103/PhysRev.56.978
-
Devesa S., Rooney A.P., Graça M.P., Cooper D., Costa L.C. Williamson-hall analysis in estimation of crystallite size and lattice strain in Bi1.34Fe0.66Nb1.34O6.35 prepared by the sol-gel method. Materials Science & Engineering B, 2021, vol. 263, pp. 114830. https://doi.org/10.1016/j.mseb.2020.114830
-
Mustapha S., Ndamitso M., Abdulkareem A.S., Tijani J.O., Shuaib D.T., Mohammed A.K., Sumaila A. Comparative study of crystallite size using Williamson-Hall and Debye-Scherrer plots for ZnO nanoparticles. Advances in Natural Sciences: Nanoscience and Nanotechnology, 2019, vol. 10, no. 4, pp. 045013. https://doi.org/10.1088/2043-6254/ab52f7
-
Leontyev I.N., Kuriganova A.B., Allix M., Rakhmatullin A., Timoshenko P.E., Maslova O.A., Mikheykin A.S., Smirnova N.V. On the evaluation of the average crystalline size and surface area of platinum catalyst nanoparticles. Physica Status Solidi (B), 2018, vol. 255, no. 10, pp. 1800240. https://doi.org/10.1002/pssb.201800240
-
Bokuniaeva A.O., Vorokh A.S. Estimation of particle size using the Debye equation and the Scherrer formula for polyphasic TiO2 powder. Journal of Physics: Conference Series, 2019, vol. 1410, no. 1, pp. 012057. https://doi.org/10.1088/1742-6596/1410/1/012057
-
Sgibnev Y.M., Nikonorov N.V., Vasilev V.N., Ignatiev A.I. Optical gradient waveguides in photo-thermo-refractive glass formed by ion exchange method. Journal of Lightwave Technology, 2015, vol. 33, no. 17, pp. 3730–3735. https://doi.org/10.1109/JLT.2015.2456239
-
Sgibnev E.M., Ignatiev A.I., Nikonorov N.V., Efimov A.M., Postnikov E.S. Effects of silver ion exchange and subsequent treatments on the UV–VIS spectra of silicate glasses. I. Undoped, CeO2-doped, and (CeO2+Sb2O3)-codoped photo-thermo-refractive matrix glasses. Journal of Non-Crystalline Solids, 2013, vol. 378, pp. 213–226. https://doi.org/10.1016/j.jnoncrysol.2013.07.010
-
Findakly V. Glass waveguides by ion exchange: a review. Optical Engineering, 1985, vol. 24, no. 2, pp. 244–250. https://doi.org/10.1117/12.7973463
-
Miao X., Ji Z., Wu J., Shen X., Wang J., Kong L., Liu M., Song C. g-C3N4/AgBr nanocomposite decorated with carbon dots as a highly efficient visible-light-driven photocatalyst. Journal of Colloid and Interface Science, 2017, vol. 502, pp. 24–32. https://doi.org/10.1016/j.jcis.2017.04.087
-
Xie R., Zhang L., Xu H., Zhong Y., Sui X., Mao Z. Fabrication of Z-scheme photocatalyst Ag-AgBr@Bi20TiO32 and its visible-light photocatalytic activity for the degradation of isoproturon herbicide. Journal of Molecular Catalysis A: Chemical, 2015, vol. 406, pp. 194–203. https://doi.org/10.1016/j.molcata.2015.05.028
-
Kuai L., Geng B., Chen X., Zhao Y., Luo Y. Facile subsequently light-induced route to highly efficient and stable sunlight-driven Ag-AgBr plasmonic photocatalyst. Langmuir, 2010, vol. 26, no. 24, pp. 18723–18727. https://doi.org/10.1021/la104022g
-
Nikonorov N.V., Evstropev S.K. Optical Materials Science. Fundamentals of Optical Glass Strength. Tutorial. St. Petersburg, SPbSU ITMO, 2009, 102 p. (in Russian)