Menu
Publications
2024
2023
2022
2021
2020
2019
2018
2017
2016
2015
2014
2013
2012
2011
2010
2009
2008
2007
2006
2005
2004
2003
2002
2001
Editor-in-Chief
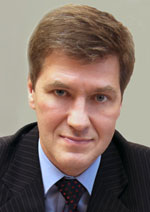
Nikiforov
Vladimir O.
D.Sc., Prof.
Partners
doi: 10.17586/2226-1494-2022-22-5-818-823
Influence of the dimension, geometry, and orientation of nanostructures on the distribution of the electric field in matters of enhancing of Raman scattering
Read the full article

Article in Russian
For citation:
Abstract
For citation:
Matveeva K.I., Zyubin A.Yu., Kon I.I., Samusev I.G. Influence of the dimension, geometry, and orientation of nanostructures on the distribution of the electric field in matters of enhancing of Raman scattering. Scientific and Technical Journal of Information Technologies, Mechanics and Optics, 2022, vol. 22, no. 5, pp. 818–823 (in Russian). doi: 10.17586/2226-1494-2022-22-5-818-823
Abstract
The presented work reflects and analyzes the results of numerical simulation of the electric field strength dependence on the dimensionality and geometry of gold nanostructures as well as their orientation relative to the polarization of the incident radiation. The simulation of the electric field strength near a single nanostructure was performed using the Finite-Difference Time-Domain (FDTD) method. FDTD modeling is an effective theoretical way to study the interaction between electromagnetic waves and plasmonic nanoobjects represented by single gold nanorods with different geometry (cylinder, spherical cylinder, elongated ellipsoid) and variable half-length (L =10–120 nm). The radiation source was a plane-polarized wave (λ = 632 nm). As a result of the simulation, the localization of the electric field near the nanostructures has been determined and the maximum theoretical values of the near-field strength have been obtained. The dependence of the electric field strength maxima has been demonstrated to be nonlinear on the half-length of gold nanostructures in case of their longitudinal orientation to E-vector. The obtained theoretical calculations of the electric field strength near the plasmonic nanoparticles, including gold nanorods, allow us to determine the optimal nanoparticle parameters and excitation conditions for the plasmon resonance occurrence which in turn provides an opportunity to create SERS-structures with a high Raman scattering enhancement.
Keywords: FDTD simulation, plasmon resonance, nanoparticles, nanorods, SERS
Acknowledgements. The research was carried out within the state assignment of Ministry of Science and Higher Education of the Russian Federation No. 75-02-2022-872.
References
Acknowledgements. The research was carried out within the state assignment of Ministry of Science and Higher Education of the Russian Federation No. 75-02-2022-872.
References
-
Brode W.R. Chemical Spectroscopy. John Wiley & Sons, 1939.
-
Sahu R.K., Mordechai S. Spectroscopic techniques in medicine: The future of diagnostics. Applied Spectroscopy Reviews, 2016, vol. 51, no. 6, pp. 484–499. https://doi.org/10.1080/05704928.2016.1157809
-
Xia J., Zhu F., Bounds J., Aluauee E., Kolomenskii A., Dong Q., He J., Meadows C., Zhang S., Schuessler H. Spectroscopic trace gas detection in air-based gas mixtures: Some methods and applications for breath analysis and environmental monitoring. Journal of Applied Physics, 2022, vol. 131, no. 22, pp. 220901. https://doi.org/10.1063/5.0091263
-
De Bleye C., Chavez P.-F., Mantanus J., Marini R., Hubert Ph., Rozet E., Ziemons E. Critical review of near-infrared spectroscopic methods validations in pharmaceutical applications. Journal of Pharmaceutical and Biomedical Analysis, 2012, vol. 69, pp. 125–132. https://doi.org/10.1016/j.jpba.2012.02.003
-
Khlebtsov N.G. Optics and biophotonics of nanoparticles with a plasmon resonance. Quantum Electronics, 2008, vol. 38, no. 6, pp. 504–529. https://doi.org/10.1070/QE2008v038n06ABEH013829
-
Boginskaya I., Gainutdinova A., Gusev A., Mailyan K., Mikhailitsyn A., Sedova M., Vdovichenko A., Ryzhikov I., Chvalun S., Lagarkov A. Detection of organic substances by a sers method using a special ag-poly(chloro-p-xylylene)-ag sandwich substrate. Coatings, 2020, vol. 10, no. 8, pp. 799. https://doi.org/10.3390/coatings10080799
-
Aitekenov S., Sultangaziyev A., Abdirova P., Yussupova L., Gaipov A., Utegulov Z., Bukasov R. Raman, Infrared and Brillouin spectroscopies of biofluids for medical diagnostics and for detection of biomarkers. Critical Reviews in Analytical Chemistry, 2022. in press. https://doi.org/10.1080/10408347.2022.2036941
-
Mandal P., Tewari B.S. Progress in surface enhanced Raman scattering molecular sensing: A review. Surfaces and Interfaces, 2022, vol. 28, pp. 101655. https://doi.org/10.1016/j.surfin.2021.101655
-
Mosier-Boss P.A. Review of SERS substrates for chemical sensing. Nanomaterials, 2017, vol. 7, no. 6, pp. 142. https://doi.org/10.3390/nano7060142
-
Goncharov V.K., Kozadaev K.V., Mel’nikov K.A., Mikitchuk E.P., Novikov A.G. Atmospheric laser deposition of sers-active colloids of noble metals (Ag, Au, and Pt). Journal of Engineering Physics and Thermophysics, 2018, vol. 91, no. 5, pp. 1291–1295. https://doi.org/10.1007/s10891-018-1860-6
-
Cao Y., Zhang J., Yang Y., Huang Z., Long N.V., Fu C. Engineering of SERS substrates based on noble metal nanomaterials for chemical and biomedical applications. Applied Spectroscopy Reviews, 2015, vol. 50, no. 6, pp. 499–525. https://doi.org/10.1080/05704928.2014.923901
-
Procházka M. Surface-Enhanced Raman Spectroscopy. Biological and Medical Physics, Biomedical Engineering, Springer, 2016, 221 p. https://doi.org/10.1007/978-3-319-23992-7
-
Zeng Z., Liu Y., Wei J. Recent advances in surface-enhanced Raman spectroscopy (SERS): Finite-difference time-domain (FDTD) method for SERS and sensing applications. TrAC Trends in Analytical Chemistry, 2016, vol. 75, pp. 162–173. https://doi.org/10.1016/j.trac.2015.06.009
-
Hao F., Nordlander P. Efficient dielectric function for FDTD simulation of the optical properties of silver and gold nanoparticles. Chemical Physics Letters, 2007, vol. 446, no. 1-3, pp. 115–118. https://doi.org/10.1016/j.cplett.2007.08.027
-
Yang Z.L., Li Q., Ruan X., Li Z., Ren B., Xu H., Tian Z. FDTD for plasmonics: Applications in enhanced Raman spectroscopy. Chinese Science Bulletin, 2010, vol. 55, no. 24, pp. 2635–2642. https://doi.org/10.1007/s11434-010-4044-0
-
Schneider J.B. Understanding the Finite-Difference Time-Domain Method. School of electrical engineering and computer science Washington State University, 2010.
-
Rafiee M., Chandra S., Ahmed H., McCormack S.J. Optimized 3D finite-difference-time-domain algorithm to model the plasmonic properties of metal nanoparticles with near-unity accuracy. Chemosensors, 2021, vol. 9, no. 5, pp. 114. https://doi.org/10.3390/chemosensors9050114
-
Grand J., Adam P.-M., Grimault A.-S., Vial A., Lamy de la Chapelle M., Bijeon J.-L., Kostcheev S., Royer P. Optical extinction spectroscopy of oblate, prolate and ellipsoid shaped gold nanoparticles: experiments and theory. Plasmonics, 2006, vol. 1, no. 2-4, pp. 135–140. https://doi.org/10.1007/s11468-006-9014-7
-
Xu J., Kvasnička P., Idso M., Jordan R.W., Gong H., Homola J., Yu Q. Understanding the effects of dielectric medium, substrate, and depth on electric fields and SERS of quasi-3D plasmonic nanostructures. Optics express, 2011, vol. 19, no. 21, pp. 20493–20505. https://doi.org/10.1364/OE.19.020493
-
Ladutenko K.S., Belov P.A. Nanophotonics integrated circuits simulation: FDTD method. Nanosystems: Physics, Chemistry, Mathematics, 2012, vol. 3, no. 5, pp. 44–64. (in Russian)
-
Cheng Y., Chen G., Wang X.-H., Yang S. Analytical investigations on FDTD numerical dispersion. Proc. of the IEEE MTT-S International Conference on Numerical Electromagnetic and Multiphysics Modeling and Optimization (NEMO), 2020, pp. 9343614. https://doi.org/10.1109/NEMO49486.2020.9343614
-
Ross M.B., Blaber M.G., Schatz G.C. Using nanoscale and mesoscale anisotropy to engineer the optical response of three-dimensional plasmonic metamaterials. Nature Communications, 2014, vol. 5, pp. 4090. https://doi.org/10.1038/ncomms5090
-
Bedics M.A., Kearns H., Cox J.M., Mabbott S., Ali F., Shand N.C., Faulds K., Benedict J.B., Graham D., Detty M.R. Extreme red shifted SERS nanotags. Chemical Science, 2015, vol. 6, no. 4, pp. 2302–2306. https://doi.org/10.1039/C4SC03917C
-
Kon I.I., Zyubin A.Y., Seteikin A.Y., Samusev I.G. FDTD simulations field on gold nanoparticles and silver nanorods. Proceedings of SPIE, 2021, vol. 11904, pp. 119041I. https://doi.org/10.1117/12.2602518
-
Zeng Z., Quan X., Yu X., Chen S., Zhang S. Nanoscale lightning rod effect in 3D carbon nitride nanoneedle: Enhanced charge collection and separation for efficient photocatalysis. Journal of Catalysis, 2019, vol. 375, pp. 361–370. https://doi.org/10.1016/j.jcat.2019.06.019
-
Nicoletti O., Wubs M., Mortensen N.A., Sigle W., van Aken P.A., Midgley P.A. Surface plasmon modes of a single silver nanorod: an electron energy loss study. Optics Express, 2011, vol. 19, no. 16, pp. 15371–15379. https://doi.org/10.1364/OE.19.015371