Menu
Publications
2025
2024
2023
2022
2021
2020
2019
2018
2017
2016
2015
2014
2013
2012
2011
2010
2009
2008
2007
2006
2005
2004
2003
2002
2001
Editor-in-Chief
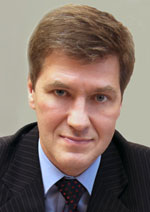
Nikiforov
Vladimir O.
D.Sc., Prof.
Partners
doi: 10.17586/2226-1494-2022-22-5-992-998
On the possibility of expanding the studied dynamic ranges in thermal anemometry
Read the full article

Article in Russian
For citation:
Abstract
For citation:
Khodunkov V.P. On the possibility of expanding the studied dynamic ranges in thermal anemometry. Scientific and Technical Journal of Information Technologies, Mechanics and Optics, 2022, vol. 22, no. 5, pp. 992–998 (in Russian). doi: 10.17586/2226-1494-2022-22-5-992-998
Abstract
The paper presents the results of a study of the possibility of expanding the dynamic ranges of hot-wire methods. The relevance of the work is due to the demand in new methods for measuring the thermal and hydrodynamic parameters of high-speed gas flows for the purposes of scientific instrumentation. The novelty of the presented technical solution lies in the use of two hot-wires anemometers with significantly different thermal inertia for simultaneous measurement of the flow velocity and the heat transfer coefficient in it. The theoretical basis of the proposed method is based on the phenomenon of the inertia of the response of any thermodynamic system under a stepwise thermal effect on it. The method consists in placing in the investigated flow two bodies of the same shape and size which have significantly different thermal inertia. The bodies are subjected to a stepped thermal effect, and the non-stationary temperature delay of the bodies relative to each other is recorded. Using the maximum value of the temperature delay, the value of the heat transfer coefficient of the bodies with the investigated flow can be evaluated by calculation. The flow rate is found from the value of the moment of time corresponding to the maximum temperature delay while using the calibration characteristic previously obtained on the reference flow. The new thermal anemometry method is scientifically substantiated, the measurement equation of the method is obtained, the measurement algorithm and the generalized scheme of the device implementing the method are developed, and the value of the expected uncertainty of the measurement results is given. The simulation results showed that the relative uncertainty provided by the presented method does not exceed 1.5 %. The developed method makes it possible to significantly increase the accuracy and expand the studied dynamic ranges of the required values for a wide range of gas flows. The method can be used in the flow measurement of gas-air flows.
Keywords: thermal anemometry, gas flow, thermal inertia, speed, heat transfer coefficient, step action, overheating, delay, signal
References
References
-
Handbuch der industriellen Messtechnik. Ed. by P. Profos. Essen, Vulkan-Verlag, 1987.
-
Pomanchenko A.F., Razdymakho S.V. Method of thermal and anemometric measurements. Patent RU 2217765. 2003. (in Russian)
-
Kuper V.J. Rubtsov M.G., Khozinskij E.F., Shamikhin A.N. Method of measuring of a gas or liquid flow speed. Patent RU 2267790. 2006. (in Russian)
-
Pomanchenko A.F. A method for measuring the parameters of the gas and liquid medium.Patent 637676. 1978. (in Russian)
-
Korzenev A.N., Garanin A.V. Method of determination of velocity and temperature of gas stream and device for its realization. Patent RU 2347227. 2009. (in Russian)
-
Khamshah N., Abdalla A.N., Koh S.P., Rashag H.F. Issues and temperature compensation techniques for hot wire thermal flow sensor: A review. International Journal of the Physical Sciences, 2011, vol. 6(14), pp. 3270–3278. https://doi.org/10.5897/IJPS11.630
-
Morris S.C., Foss J.F. Transient thermal response of a hot-wire anemometer. Measurement Science and Technology, 2003, vol. 14, no. 3, pp. 251–259. https://doi.org/10.1088/0957-0233/14/3/302
-
Palma J., Labbé R. Rugged constant-temperature thermal anemometer. Review of Scientific Instruments, 2016, vol. 87, no. 12, pp. 125112. http://dx.doi.org/10.1063/1.4972585
-
Kunkel G.J., Arnold C.B., Smits A.J. Development of NSTAP: nanoscale thermal anemometry probe. Collection of Technical Papers - 36th AIAA Fluid Dynamics Conference. Vol. 3, 2006, pp. 1938–1945. https://doi.org/10.2514/6.2006-3718
-
Fu M.K., Fan Y., Hultmark M. Design and validation of a nanoscale cross-wire probe (X-NSTAP). Experiments in Fluids, 2019, vol. 60, no. 6, pp. 99. https://doi.org/10.1007/s00348-019-2743-0
-
ValiullinR., SharafutdinovR., Fedotov V., Ramazanov A., Vakhitova G., Kosmylin D. A new radial-azimuth thermal anemometer for determining the direction and velocity of fluid flow in the wellbore. Proc. of the SPE Russian Petroleum Technology Conference (RPTC), 2018, pp. SPE-191565-18RPTC-MS. https://doi.org/10.2118/191565-18RPTC-MS
-
Grek G.R., Boyko A.V., Gilyov V.M., Zverkov I.D., Sorokin A.M. The automated system of hot-wire anemometer information acquisition in the aerophysical experiment. International Journal of Applied and Fundamental Research, 2014, no. 5-1, pp. 11–14. (in Russian)
-
Ligęza P. Advanced temperature compensation and correction techniques in thermal anemometry. Instrumentation Science & Technology, 2015, vol. 43, no. 1, pp. 21–43. https://doi.org/10.1080/10739149.2014.942915
-
Arlit M., Schleicher E., Hampel U. Thermal anemometry grid sensor. Sensors (Basel), 2017, vol. 17, no. 7, pp. 1663. https://doi.org/10.3390/s17071663
-
Kondratev G.M. Regular Thermal Conditions. Moscow, Gostehizdat Publ., 1954, 408 p. (in Russian)
-
Dulnev G.N. Theory of Heat and Mass Transfer. Tutorial. St. Petersburg, NIU ITMO, 2012, 195 p.(in Russian)