Menu
Publications
2025
2024
2023
2022
2021
2020
2019
2018
2017
2016
2015
2014
2013
2012
2011
2010
2009
2008
2007
2006
2005
2004
2003
2002
2001
Editor-in-Chief
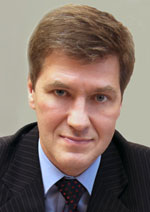
Nikiforov
Vladimir O.
D.Sc., Prof.
Partners
doi: 10.17586/2226-1494-2022-22-5-832-838
Implementation of digital holographic interferometry for pulsed plasma studies
Read the full article

Article in Russian
For citation:
Abstract
For citation:
Kozhevnikova A.M., Ivankov A.S., Schitz D.V., Alekseenko I.V. Implementation of digital holographic interferometry for pulsed plasma studies. Scientific and Technical Journal of Information Technologies, Mechanics and Optics, 2022, vol. 22, no. 5, pp. 832–838 (in Russian). doi: 10.17586/2226-1494-2022-22-5-832-838
Abstract
The study of low-temperature plasma generated in pulsed mode at atmospheric pressure was carried out. The purpose of the presented research is to develop a method of digital holographic interferometry for registration and evaluation of parameters of low-temperature plasma at atmospheric pressure in pulsed mode. This type of plasma is currently applied in medicine and biology. Thus, there is a need to control the exposure dose and plasma environment formation regimes. As plasma parameters, it can be considered its electron concentration which can be calculated through the estimation of the refractive index of plasma pulse in relation to unperturbed state. The plasma pulses were activated in Helium. The plasma pulse frequency was 5 kHz and its duration was 750 ns. During an investigation a laboratory set-up for recording holographic images of plasma pulses was developed. Holograms are acquired on a digital camera and a pulsed laser INNOLAS SpitLight Hybrid II at a wavelength of 532 nm with pulse duration of 10 ns is used as a source of coherent radiation. In order to record plasma pulses, the laser, plasma generator and digital camera were strictly synchronized to each other. During the experiment, a series of about 500 holograms were acquired, and the reconstruction of the phase of the object field was calculated. Analysis of the sequence of holograms allowed calculations of phase difference (interferograms) related to the refractive index of low-temperature pulsed plasma in Helium. It is known that low-temperature plasma leads to low phase delay which forms low phase contrast of the evaluated interferograms. For this purpose, we carried out preliminary experiments with plasma-arc that has similar temporary parameters, however, with a higher phase contrast of the interferograms. The paper presents experimental results obtained by studying the phase contrast of the refractive index of pure Helium, plasma-arc and plasma pulses in Helium. Thus, the effectiveness of both the experimental set-up and the method to evaluate the interferograms related to the refractive index of the plasma pulse was verified. The data obtained can then be used to estimate the electron concentration of the plasma. However, it needs to increase the sensitivity of the method in order to enhance phase contrast. Increase of sensitivity can be done by means of extension of the spectral range, for example, toward to infra-red.
Keywords: digital holographic interferometry, holographic interferometry, nonthermal plasma, plasma electron concentration, experiment automation
Acknowledgements. This study was performed in accordance with the Government Assignment supported by the RF Ministry of education and science, Project No. FZWM-2020-0003 “Investigation of advanced materials and methods for plasma and photo-therapy of oncological and skin diseases and septic complications” for the years 2020–2023.
References
Acknowledgements. This study was performed in accordance with the Government Assignment supported by the RF Ministry of education and science, Project No. FZWM-2020-0003 “Investigation of advanced materials and methods for plasma and photo-therapy of oncological and skin diseases and septic complications” for the years 2020–2023.
References
1. Weltmann K.D., Kindel E., von Woedtke T., Hähnel M., Stieber M., Brandenburg R. Atmospheric-pressure plasma sources: Prospective tools for plasma medicine. Pure and Applied Chemistry, 2010, vol. 82, no. 6, pp. 1223–1237. https://doi.org/10.1351/PAC-CON-09-10-35
2. Sosnin E.A., Stoffels E., Erofeev M.V., Kieft I.E., Kunts S.E. The effects of UV irradiation and gas plasma treatment on living mammalian cells and bacteria: a comparative approach. IEEE Transactions on Plasma Science, 2004, vol. 32, no. 4, pp. 1544–1550. https://doi.org/10.1109/TPS.2004.833401
3. Zhou Q., Cheng C., Meng Y. Electron density and temperature measurement by stark broadening in a cold argon arc-plasma jet at atmospheric pressure. Plasma Science and Technology, 2009, vol. 11, no. 5, pp. 560–563. https://doi.org/10.1088/1009-0630/11/5/09
4. Vest C.M. Holographic Interferometry. John Wiley&Sons, 1979, pp. 387–396.
5. Schnars U., Juptner W. Digital Holography. Springer-Verlag and Heidelberg GmbH & Company K, 2005, 164 p. https://doi.org/10.1007/b138284
6. Kreis T. Handbook of holographic interferometry: optical and digital methods. John Wiley & Sons, 2006, pp. 379.
7. Ostrovskaya G.V., Ostrovsky Y.I. IV Holographic methods of plasma diagnostics. Progress in Optics, 1985, vol. 22, pp. 197–270. https://doi.org/10.1016/S0079-6638(08)70150-1
8. Zaidel’ A.N. Application of holographic interferometry for plasma diagnostics. Soviet Physics – Uspekhi, 1986, vol. 29, no. 5, pp. 447–466. https://doi.org/10.1070/PU1986v029n05ABEH003381
9. Khanzadeh M., Jamal F., Shariat M. Experimental investigation of gas flow rate and electric field effect on refractive index and electron density distribution of cold atmospheric pressure-plasma by optical method, Moiré deflectometry. Physics of Plasmas, 2018, vol. 25, no. 4, pp. 043516. https://doi.org/10.1063/1.5018054
10. Gusev M.E., Alexeenko I.V. Methods of digital stroboholographic interferometry in investigation of mechanical vibrations. Izvestija vysshih uchebnyh zavedenij. Fizika, 2015, vol. 58, no. 11-3, pp. 10–17. (in Russian)
11. Takeda M., Ina H., Kobayashi S. Fourier-transform method of fringe-pattern analysis for computer-based topography and interferometry. Journal of the Optical Society of America, 1982, vol. 72, no. 1, pp. 156–160. https://doi.org/10.1364/JOSA.72.000156