Menu
Publications
2025
2024
2023
2022
2021
2020
2019
2018
2017
2016
2015
2014
2013
2012
2011
2010
2009
2008
2007
2006
2005
2004
2003
2002
2001
Editor-in-Chief
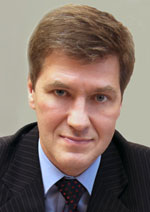
Nikiforov
Vladimir O.
D.Sc., Prof.
Partners
doi: 10.17586/2226-1494-2022-22-6-1037-1047
Modelling of a composite waveguide holographic display
Read the full article

Article in Russian
For citation:
Abstract
For citation:
Kharitonov D.Yu., Akhmetov D.M., Muslimov E.R., Gilfanov A.R., Pavlycheva N.K. Modelling of a composite waveguide holographic display. Scientific and Technical Journal of Information Technologies, Mechanics Optics, 2022, vol. 22, no. 6, pp. 1037–1047 (in Russian). doi: 10.17586/2226-1494-2022-22-6-1037-1047
Abstract
Optical designs of waveguide-type augmented reality displays are investigated. Displays based on volume phase holograms are notable for their small size, large exit pupil and high transmittance both in the projected image channel and in the direct vision channel. However, with an increase of the aperture, field of view and working spectral range, the spread of the values of the beam angle of incidence and the wavelength increases when solving the diffraction problem at different points on the hologram surface which imposes restrictions on spatial resolution and diffraction efficiency. To overcome this phenomenon, it is proposed to use a composite hologram which represents a volume phase grating divided into zones with independently varying parameters of the fringes tilt, their shape, the holographic layer thickness and the refraction index modulation depth. We propose an algorithm that allows ray tracing through a hologram recorded by two coherent point sources using an auxiliary aspherical mirror. The initial ray tracing in the hologram recording scheme is performed using the error function minimization by the orthogonal descent and golden section methods. Based on the results obtained, the directional vectors of the diffracted beam are calculated using the Welford equation. Using the tracing results, the hologram diffraction efficiency is computed with the Kogelnik’s coupled wave theory. The proposed algorithms are implemented in the Zemax Optics Studio software. The application of the proposed composite hologram element and the tools for operation modeling are shown on an example of display operating in the range of 510–530 nm with the field of view of 7°36ʹ × 5°48ʹ and the exit pupil diameter of 8 mm. It is shown that the proposed solutions make it possible to increase the diffraction efficiency by 3.45 times. At the same time, the spatial resolution increases by 12.7 % varying across the field of view in the range of 0ʹ44ʺ–1ʹ6ʺ. The use of composite holograms allows one to create displays with higher spatial resolution and brightness of the projected image as well as uniformity of the characteristics across the field of view.
Keywords: diffraction efficiency, volume phase hologram, waveguide holographic display, augmented reality
Acknowledgements. The research is funded by the Russian Science Foundation grant No. 21-79-00082. We also would like to thank our colleague Ilya A. Guskov for his help.
References
Acknowledgements. The research is funded by the Russian Science Foundation grant No. 21-79-00082. We also would like to thank our colleague Ilya A. Guskov for his help.
References
-
Cakmakci O., Rolland J.P. Head-worn displays: A review.Journal of Display Technology, 2016, vol. 2, no. 3, pp. 199–217. https://doi.org/10.1109/JDT.2006.879846
-
Kress B.C. Optical waveguide combiners for AR headsets: features and limitations. Proceedings of SPIE, 2019, vol. 11062, pp. 110620J. https://doi.org/10.1117/12.2527680
-
Amitai Y., Friesem A.A., Weiss V. Holographic elements with high efficiency and low aberrations for helmet displays. Applied Optics, 1989, vol. 28, no. 16, pp. 3405–3416. https://doi.org/10.1364/AO.28.003405
-
Yoshida T., Tokuyama K., Takai Y., Tsukuda D., Kaneko T., Suzuki N., Anzai T., Yoshikaie A., Akutsu K., Machida A. A plastic holographic waveguide combiner for light-weight and highly‐transparent augmented reality glasses. Journal of the Society for Information Display, 2018, vol. 26, no. 5, pp. 280–286. https://doi.org/10.1002/jsid.659
-
Mukawa H., Akutsu K., Matsumura I., Nakano S., Yoshida T., Kuwahara M., Aiki K. A full-color eyewear display using planar waveguides with reflection volume holograms.Journal of the Society for Information Display, 2009, vol. 17, no. 3, pp. 185–193. https://doi.org/10.1889/JSID17.3.185
-
Muslimov E.R., Pavlycheva N.K., Guskov I.A. Concept of composite holographic optical elements. Photonics Russia, 2020, vol. 14, no. 7, pp. 586–599. (in Russian). https://doi.org/10.22184/1993-7296.FRos.2020.14.7.586.599
-
Muslimov E., Pavlycheva N., Guskov I., Akhmetov D., Kharitonov D.Yu. Spectrograph with a composite holographic dispersive element. Proceedings of SPIE, 2021, vol. 11871, pp. 187198. https://doi.org/10.1117/12.2596922
-
Welford W. A vector raytracing equation for hologram lenses of arbitrary shape. Optics Communications, 1975, vol. 14, no. 3, pp. 322–323. https://doi.org/10.1016/0030-4018(75)90327-2
-
Grebennikova I.V. Optimization Methods. Yekaterinburg, UrFU, 2017, 148 с. (in Rusian)
-
Gasnikov A.V. Modern Numerical Methods of Optimization. Universal Gradient Descent Method. Moscow, MIPT, 2018, 181 p. (in Russian)
-
Kogelnik H. Coupled wave analysis for thick hologram gratings. The Bell System Technical Journal, 1969, vol. 48, no. 9, pp. 2909–2947. https://doi.org/10.1002/j.1538-7305.1969.tb01198.x
-
Moharam M.G., Grann E.B., Pommet D.A., Gaylord T.K. Formulation for stable and efficient implementation of the rigorous coupled-wave analysis of binary gratings. Journal of the Optical Society of America A: Optics and Image Science, and Vision, 1995, vol. 12, no. 5, pp. 1068–1076. https://doi.org/10.1364/JOSAA.12.001068
-
Romanova G.E., Nguyen N.S. Aberration analysis of a wedge as a compensator element in augmented and virtual reality systems. Scientific and Technical Journal of Information Technologies, Mechanics and Optics,2021, vol. 21, no. 6, pp. 808–816. (in Russian). https://doi.org/10.17586/2226-1494-2021-21-6-808-816
-
Ivaniuk A.A. Optical module design for augmented reality glasses. Scientific and Technical Journal of Information Technologies, Mechanics and Optics, 2020, vol. 20, no. 5,pp. 642–648. (in Russian). https://doi.org/10.17586/2226-1494-2020-20-5-642-648
-
Romanova G.E., Koreshev S.N., Sidorenko V.S. Calculation and modeling of the augmented reality optical-waveguide structure based on holographic elements. HOLOEXPO 2019: XVI International Conference on Holography and Applied Optical Technology. Proceeedings, 2019, pp. 164–167. (in Russian)
-
Grad Ya.A., Nikolaev V.V., Odinokov S.B., Solomashenko A.B. Augmented reality indicator based on a lightguide plate with transmissive DOEs. Holography. Science and Practice XIV International Conference HOLOEXPO 2017. Proceedings, 2017, pp. 133–137. (in Russian)
-
Peixoto C., Valentim P.T., Sousa P.C., Dias D., Araújo C., Pereira D., Machado C.F., Pontes A.J., Santos H., Cruz S. Injection molding of high-precision optical lenses: A review. Precision Engineering, 2022, vol. 76, pp. 29–51. https://doi.org/10.1016/j.precisioneng.2022.02.002
-
Waldern J.D., Grant A.J., Popovich M.M. DigiLens switchable Bragg grating waveguide optics for augmented reality applications. Proceedings of SPIE, 2018, vol. 10676, pp. 106760G. https://doi.org/10.1117/12.2315719
-
Angervaks A.E., Muravev N.V., Borisov V.N., Okun R.A., Vostrikov G.N., Popov M.V. Waveguide with segmented diffraction optical elements and near-eye display. Patent RU2752556C1, 2021. (in Russian)
-
Lalanne P., Hugonin J.-P. High-order effective-medium theory of subwavelength gratings in classical mounting: application to volume holograms. Journal of the Optical Society of America A: Optics and Image Science, and Vision, 1998, vol. 15, no. 7, pp. 1843–1851. https://doi.org/10.1364/JOSAA.15.001843
-
Gerritsen H.J., Thornton D.K., Bolton S.R. Application of Kogelnik’s two-wave theory to deep, slanted, highly efficient, relief transmission gratings. Applied Optics, 1991, vol. 30, no. 7, pp. 807–814. https://doi.org/10.1364/AO.30.000807
-
Moharam M.G., Young L. Criterion for Bragg and Raman-Nath diffraction regimes. Applied Optics, 1978, vol. 17, no. 11, pp. 1757–1759. https://doi.org/10.1364/AO.17.001757