Menu
Publications
2024
2023
2022
2021
2020
2019
2018
2017
2016
2015
2014
2013
2012
2011
2010
2009
2008
2007
2006
2005
2004
2003
2002
2001
Editor-in-Chief
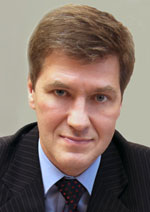
Nikiforov
Vladimir O.
D.Sc., Prof.
Partners
doi: 10.17586/2226-1494-2022-22-6-1197-1204
Simulation of radiative transfer in gas-liquid foams
Read the full article

Article in Russian
For citation:
Abstract
For citation:
Isaeva A.A., Isaeva E.A., Pantyukov A.V. Simulation of radiative transfer in gas-liquid foams. Scientific and Technical Journal of Information Technologies, Mechanics and Optics, 2022, vol. 22, no. 6, pp. 1197–1204 (in Russian).
doi: 10.17586/2226-1494-2022-22-6-1197-1204
Abstract
The results of the radiation light transfer in the gas-liquid foams are presented. To study the probing light transfer in gas-liquid foam-like media at different stages of aging, a Monte Carlo numerical simulation method is proposed. To take into account the re-reflections at the phase interfaces, the approach based on the Fresnel formula is applied. Kelvin cells structures are considered as a model medium, imitating gas bubbles in a liquid matrix during aging (coarsening), which represents the transition of a foam-like medium from “wet” to “dry”. Such transition is caused by the slow liquid flow along the gas cells walls and Plateau-Gibbs channels due to the influence of the gravitational force in an isolated system. During the evolution process, the volume fraction of the liquid phase decreases and the average size of the gas cells of the foam-like medium increases. The three-dimensional cellular structure at different evolution stages is represented as a system of close-packed ordered spheres or tetradecahedrons of various geometric sizes depending on the aging process duration. The Monte Carlo modeling of the radiation light transfer in scattering two-phase foam-like media, taking into account the redistribution at the interfaces of two phases by use of Fresnel formulas at different coursing stages, is presented. The transmittance and backscattering coefficients of a probing light are obtained using the Monte Carlo numerical simulations and Fresnel formulas for the gas-liquid foams. The obtained results are well correlated with the spectrometric measurements of the foamed liquid produced by Gillete (Gillete shaving cream). The influence of the anisotropy factor on the optical length of the probing light was carried out. The development of the theoretical approaches to the diagnostics of the two-phase foam-like materials makes it possible to synthesize foamed materials with established rheological and structural properties to increase and improve the efficiency of evaluating of the functional characteristics for such materials.
Keywords: gas-liquid foams, Kelvin cell, transmission coefficient, Monte Carlo simulation, Fresnel formulas
Acknowledgements. This work was supported by the Russian Science Foundation (project No. 21-79-00051).
References
Acknowledgements. This work was supported by the Russian Science Foundation (project No. 21-79-00051).
References
-
Li N., Fu Y., Lu Q., Xiao C. Microstructure and performance of a porous polymer membrane with a copper nano-layer using vapor-induced phase separation combined with magnetron sputtering. Polymers, 2017, vol. 9, no. 10, pp. 524–527. https://doi.org/10.3390/polym9100524
-
Poh P.S.P., Chhaya M.P., Wunner F.M., De-Juan-Pardo E.M., Schilling A.F., Schantz J.-T., van Griensven M., Hutmacher D.W. Polylactides in additive biomanufacturing. Advanced Drug Delivery Reviews, 2016, vol. 107, pp. 228–246. https://doi.org/10.1016/j.addr.2016.07.006
-
ZimnyakovD.A., YuvchenkoS.A., Isaeva A.A., Isaeva E.A., Tsypin D.V. Growth/collapse kinetics of the surface bubbles in fresh constrained foams: transition to self-similar evolution. Colloids and Surfaces A: Physicochemical and Engineering Aspects, 2019, vol. 579, pp. 123693. https://doi.org/10.1016/j.colsurfa.2019.123693
-
HollisterS.J.Porous scaffold design for tissue engineering. Nature Materials, 2005, vol. 4, no. 7, pp. 518–524. https://doi.org/10.1038/nmat1421
-
Sadovoy M.A., Larionov P.M., Samokhin A.G., Rozhnova O.M. Cellular matrices (scaffolds) for bone regeneration: state of the art. Journal of Spine Surgery, 2014, no. 2, pp. 79–86. (in Russian). https://doi.org/10.14531/ss2014.2.79-86
-
Ngadiman N.H.A., Yusof N.M., Idris A., Fallahiarezoudar E., Kurniawan D. Novel processing technique to produce three dimensional polyvinyl alcohol/maghemite nanofiber scaffold suitable for hard tissues. Polymers, 2018, vol. 10, no. 4, pp. 353. https://doi.org/10.3390/polym10040353
-
SeoS.J.,MahapatraC., Singh R.K., KnowlesJ.C., KimH.-W. Strategies for osteochondral repair: Focus on scaffolds. Journal of Tissue Engineering, 2014, vol. 5, pp. 1–5. https://doi.org/10.1177/2041731414541850
-
Hokmabad V.R., Davaran S., Ramazani A., Salehi R. Design and fabrication of porous biodegradable scaffolds: a strategy for tissue engineering. Journal of Biomaterials Science, Polymer Edition, 2017, vol. 28, no. 16, pp. 1797–1825. https://doi.org/10.1080/09205063.2017.1354674
-
Langer R., Vacanti J.P. Tissue engineering. Science, 1993, vol. 260, no. 5110, pp. 920–926. https://doi.org/10.1126/science.8493529
-
Celikkin N., Rinoldi C., Costantini M., Trombetta M., Rainer A., Święszkowski W. Naturally derived proteins and glycosaminoglycan scaffolds for tissue engineering applications. Materials Science and Engineering: C, 2017, vol. 78, pp. 1277–1299. https://doi.org/10.1016/j.msec.2017.04.016
-
Laube T., Weisser J., Berger S., Börner S., Bischoff S., Schubert H., Gajda M., Bräuer R., Schnabelrauch M. In situ foamable, degradable polyurethane as biomaterial for soft tissue repair. Materials Science and Engineering: C, 2017, vol. 78, pp. 163–174. https://doi.org/10.1016/j.msec.2017.04.061
-
O’Brien F.J. Biomaterials & scaffolds for tissue engineering. Materials Today, 2011, vol. 14, no. 3, pp. 88–95. https://doi.org/10.1016/S1369-7021(11)70058-X
-
Maitz M. Applications of synthetic polymers in clinical medicine. Biosurface and Biotribology, 2015, vol. 1, no. 3, pp. 161–176. https://doi.org/10.1016/j.bsbt.2015.08.002
-
Dehghani F., Annabi N. Engineering porous scaffolds using gas-based techniques. Current Opinion in Biotechnology, 2011, vol. 22, no. 5, pp. 661–666. https://doi.org/10.1016/j.copbio.2011.04.005
-
Quirk R.A., France R.M., Shakesheff K.M., Howdle S.M. Supercritical fluid technologies and tissue engineering scaffolds. Current Opinion in Solid State and Materials Science, 2004, vol. 8, no. 3-4, pp. 313–321. https://doi.org/10.1016/j.cossms.2003.12.004
-
Loh Q.L., Choong C. Three-dimensional scaffolds for tissue engineering applications: Role of porosity and pore size. Tissue Engineering Part B: Reviews, 2013, vol. 19, no. 6, pp. 485–502.https://doi.org/10.1089/ten.teb.2012.0437
-
Durian D.J., Weitz D.A., Pine D.J. Multiple light-scattering probes of foam structure and dynamics. Science, 1991, vol. 252, no. 5006, pp. 686–688. http://doi.org/10.1126/science.252.5006.686
-
Koehler S.A., Stone H.A., Brenner M.P., Eggers J. Dynamics of foam drainage. Physical Review E, 1998, vol. 58, no. 2, pp. 2097–2106. https://doi.org/10.1103/PhysRevE.58.2097
-
Kraynik A.M. Foam drainage. Internal Report, 1983, 83-0844.
-
Schmiedeberg M., Miri M.F., Stark H. Photon channelling in foams.European Physical Journal E, 2005, vol. 18, no. 1, pp. 123–131. http://doi.org/10.1140/epje/i2005-10034-6
-
Koehler S.A., Hilgenfeldt S., Stone H.A. A Generalized view of foam drainage: experiment and theory. Langmuir, 2000, vol. 16, no. 15, pp. 6327–6341. https://doi.org/10.1021/la9913147
-
Cantat I., Cohen-Addad S., Elias F., Graner F., Höhler R., Pitois O., Rouyer F., Saint-Jalmes A. Foams: Structure and Dynamics. Oxford, Oxford University Press, 2013, 278 p.
-
Onaka S.Superspheres: Intermediate shapes between spheres and polyhedra. Symmetry, 2012, vol. 4, no. 3, pp. 336–343.https://doi.org/10.3390/sym4030336
-
Zimnyakov D.A., Yuvchenko S.A., Isaeva A.A., Isaeva E.A., Ushakova O.V. Anisotropy of light scattering by foamed liquids. Optics and Spectroscopy, 2018, vol. 125, no. 5, pp. 795–802. https://doi.org/10.1134/S0030400X18110371