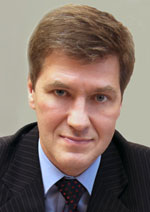
Nikiforov
Vladimir O.
D.Sc., Prof.
doi: 10.17586/2226-1494-2022-22-6-1226-1236
Modeling and simulation ofone- and two-row six-bladed ducted fans
Read the full article

For citation:
Abstract
Acknowledgements. This work was financially supported by the Ministry of Science and Higher Education of the Russian Federation in the course of the project “Fundamental bases of mechanics, control and management systems for unmanned aircraft systems with shaping structures deeply integrated with propulsion systems and unique properties not used today in manned aviation”, No. FEFM-2020-0001.
References
-
Chovancová A., Fico T., Chovanec L., Hubinský P. Mathematical modelling and parameter identification of quadrotor (a survey). Procedia Engineering, 2014, vol. 96, pp. 172–181. https://doi.org/10.1016/j.proeng.2014.12.139
-
Ostroukhov S.P. Aerodynamics of Aircraft Propellers and Annular Circular Propellers. Moscow, Fizmatlit, 2014, 328 p. (in Russian)
-
Buzikin O.G., Kazakov A.V., Shustov A.V. Numerical simulation of the aerodynamic performance of a micro air vehicle. TSAGI Science Journal. 2010, vol. 41, no. 5, pp. 535–550. https://doi.org/10.1615/TsAGISciJ.v41.i5.40
-
Nazarov D., Kondryakova A. Analisys of the flow past the screw with the use of numericaland experimental simulation. Izvestia of Samara Scientific Center of the Russian Academy of Sciences, 2018, vol. 20, no. 4-1, pp. 70–75. (in Russian)
-
Rumsey C.L., Biedron R., Farassat F., Spence P. Ducted-fan engine acoustic predictions using a Navier-Stokes code. Journal of Sound and Vibration, 1998, vol. 213, no. 4, pp. 643–664. https://doi.org/10.1006/jsvi.1998.1519
-
Reboul G., Polacsek C., Lewy S., Heib S. Aeroacoustic computation of ducted-fan broadband noise using LES data. Journal of the Acoustical Society of America, 2008, vol. 123, no. 5, pp. 3539. https://doi.org/10.1121/1.2934519
-
Myers L., Rhee W., Mclaughlin D. Aeroacoustics of vertical lift ducted rotors. Proc. of the 15th AIAA/CEAS Aeroacoustics Conference (30th AIAA Aeroacoustics Conference), 2009, pp. 2009-3333. https://doi.org/10.2514/6.2009-3333
-
Astley R., Sugimoto R., Achunche I., Kewin M., Mustafi P., Deane E. A review of CAA for fan duct propagation and radiation, with application to liner optimization. Procedia Engineering, 2010, vol. 6, pp. 143–152. https://doi.org/10.1016/j.proeng.2010.09.016
-
Malgoezar A.M., Vieira A., Snellen M., Simons D.G., Veldhuis L.L. Experimental characterization of noise radiation from a ducted propeller of an unmanned aerial vehicle. International Journal of Aeroacoustics, 2019, vol. 18, no. 4-5, pp. 372–391 https://doi.org/10.1177/1475472X19852952
-
Zhang T., Barakos G.N. Review on ducted fans for compound rotorcraft. The Aeronautical Journal, 2020, vol. 124, no. 1277, pp. 941–974. https://doi.org/10.1017/aer.2019.164
-
Akturk A., Shavalikul A., Camci C. PIV measurements and computational study of a 5-inch ducted fan for V/STOL UAV applications. Proc. of the 47th AIAA Aerospace Sciences Meeting including the New Horizons Forum and Aerospace Exposition, 2009, pp. 2009-332. https://doi.org/10.2514/6.2009-332
-
Akturk A., Camci C. Experimental and computational assessment of a ducted-fan rotor flow model. Journal of Aircraft, 2012, vol. 49, no. 3, pp. 885–897. https://doi.org/10.2514/1.C031562
-
Yilmaz S., Erdem D., Kavsaoǧlu M. Effects of duct shape on a ducted propeller performance. Proc. of the 51st AIAA Aerospace Sciences Meeting including the New Horizons Forum and Aerospace Exposition, 2013, pp. 2013–0803. https://doi.org/10.2514/6.2013-803
-
Akturk A., Camci C. Tip clearance investigation of a ducted fan used in VTOL unmanned aerial vehicles. Part I: Baseline experiments and computational validation. Journal of Turbomachinery, 2014, vol. 136, no. 2, pp. 021004. https://doi.org/10.1115/1.4023468
-
Xu H.-Y., Xing S.-L., Ye Z.-Y. Numerical study of ducted-fan lip stall suppression based on inflatable leading lip cell. Procedia Engineering, 2015, vol. 126, pp. 158–162. https://doi.org/10.1016/j.proeng.2015.11.202
-
Biava M., Barakos G.N. Optimisation of ducted propellers for hybrid air vehicles using high-fidelity CFD. The Aeronautical Journal, 2016, vol. 120, no. 1232, pp. 1632–1657. https://doi.org/10.1017/aer.2016.78
-
Chen J., Li L., Huang G., Xiang X. Numerical investigations of ducted fan aerodynamic performance with tip-jet. Aerospace Science and Technology, 2018, vol. 78, pp. 510–521. https://doi.org/10.1016/j.ast.2018.05.016
-
Ohanian O.J., Karni E.D., Londenberg W.K., Gelhausen P.A., Inman D.J. Ducted-fan force and moment control via steady and synthetic jets. Journal of Aircraft, 2011, vol. 48, no. 2, pp. 514–526
-
Mojzyh E.I., Zavalov O.A., Kuznetsov A.V. Experimental investigation of aerodynamic characteristics of unmanned aerial vehicle with lifted system of shrouded rotor. Trudy MAI, 2012, no. 50, pp. 11. (i
-
Dehaeze F., Barakos G.N. Hovering rotor computations using an aeroelastic blade model. The Aeronautical Journal, 2012, vol. 116, no. 1180, pp. 621–649. https://doi.org/10.1017/S0001924000007107
-
Abalakin I.V., Bakhvalov P.A., Bobkov V.G., Kozubskaya T.K., Anikin V.A Numerical simulation of aerodynamic and acoustic characteristics of rotor in ring. Matematicheskoe modelirovanie, 2015, vol. 27, no. 10, pp. 125–144. (in Rusian)
-
Abalakin I.V., Anikin V.A., Bakhvalov P.A., Bobkov V.G., Kozubskaya T.K., Numerical investigation of the aerodynamic and acoustical properties of a shrouded rotor. Fluid Dynamics, 2016, vol. 51, no. 3, pp. 419–433.
-
Dehaeze F., Barakos G.N., Kusyumov A.N., Kusyumov S.A., Mikhailov S.A. Exploring the detached-eddy simulation for main rotor flows. Russian Aeronautics, 2018, vol. 61, no. 1, pp. 37–44.
-
Golovkin M.A., Kochish S.I., Kritsky B.S. Calculation procedure of aerodynamic characteristics of the combined carrying system of the aircraft. Trudy MAI, 2012, no. 55, pp. 5. (in Russian)
-
Kopiev V.F., Titarev V.A., Belyaev I.V. Development of a methodology for propeller noise calculation on high-performance computer. TSAGI Science Journal, 2014, vol. 45, no. 3-4, pp. 293–327. https://doi.org/10.1615/TsAGISciJ.2014011857
-
Costes M., Renaud T., Rodriguez B. Rotorcraft simulations: a challenge for CFD. International Journal of Computational Fluid Dynamics, 2012, vol. 26, no. 6-8, pp. 383–405. https://doi.org/10.1080/10618562.2012.726710
-
Costes M., Renaud T., Rodriguez B., Reboul G. Application of vorticity confinement to rotor wake simulations. International Journal of Engineering Systems, Modelling and Simulation, 2012, vol. 4, no. 1-2, pp. 102–112.
-
Popov N.I., Emelianova O.V., Jatsun S.F. Modelling of dynamics of flight of a quadrotor helicopter. Vestnik Voronezhskogo instituta GPS MChS Rossii, 2014, no. 4(13), pp. 69–75.
-
Kanatnikov A. N., Akopyan K. R. The plane motion control of the quadrocopter. Mathematics and Mathematical Modelling, 2015, no. 2, pp. 23–36. (in Russian). https://doi.org/10.7463/mathm.0215.0789477
-
Shydakov V.I. Ground effect on aerodynamic characteristics of aerial vehicle with lifted system of shrouded rotor. Trudy MAI, 2011, no. 49, pp. 24. (in Russian)
-
Volkov K. Numerical analysis of Navier-Stokes equations on unstructured meshes. Handbook on Navier-Stokes Equations: Theory and Analysis. Nova Science, 2016, pp. 365–442.
-
Volkov K. Multigrid and preconditioning techniques in CFD applications. CFD Techniques and Thermo-Mechanics Applications. Springer International Publishing, 2018, pp. 83–149. https://doi.org/10.1007/978-3-319-70945-1_6
-
Suvorov A.S., Korotin P.I., Sokov E.M. Finite element method for simulating noise emission generated by inhomogeneities of bodies moving in a turbulent fluid flow. Acoustical Physics, 2018, vol. 64, no. 6, pp. 778–788.
-
Spalart P.R., Allmaras S.R. A one-equation turbulence model for aerodynamic flows. Proc. of the 30th Aerospace Sciences Meeting and Exhibit, 2992, pp. 1992-0439.
-
Menter F.R. Zonal two-equation k-ωturbulence models for aerodynamic flows. Proc. of the 23rd Fluid Dynamics, Plasmadynamics, and Lasers Conference, 1993, pp. 1993-2906. https://doi.org/10.2514/6.1993-2906
-
Menter F.R., Langtry R., Volker S. Transition modelling for general purpose CFD codes. Flow, Turbulence and Combustion, 2006, vol. 77, no. 1-4, pp. 277–303. https://doi.org/10.1007/s10494-006-9047-1