Menu
Publications
2025
2024
2023
2022
2021
2020
2019
2018
2017
2016
2015
2014
2013
2012
2011
2010
2009
2008
2007
2006
2005
2004
2003
2002
2001
Editor-in-Chief
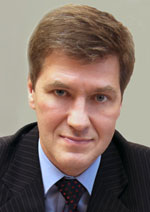
Nikiforov
Vladimir O.
D.Sc., Prof.
Partners
doi: 10.17586/2226-1494-2023-23-1-62-67
Improvement of the automatic temperature stabilisation process in the cryovacuum unit
Read the full article

Article in English
For citation:
Abstract
For citation:
Golikov O.Yu., Yerezhep D., Sokolov D.Yu. Improvement of the automatic temperature stabilisation process in the cryovacuum unit. Scientific and Technical Journal of Information Technologies, Mechanics and Optics, 2023, vol. 23, no. 1, pp. 62–67. doi: 10.17586/2226-1494-2023-23-1-62-67
Abstract
This study concerns the issues of temperature stabilization in units used to research the properties of molecules at low and ultra-low temperatures. This research is relevant due to the need to increase the speed and accuracy of the data obtained. Using the LabView graphical programming environment tools, a control program was created for the LakeShore 325 thermocontroller which reacts when the current temperature is close to the control point temperature set by the researcher. By adding controls for the heating element power and PID controller boot times, it is possible to use them more flexibly. The method was verified for the temperature control points of 40 K, 100 K, 150 K and 200 K. A comparison of the proposed temperature stabilization program with the standard PID controller solution demonstrates the advantages of the former. The speed of reaching the control points was doubled. The digitalization of the LakeShore 325 thermocontroller makes it possible to work further on improving temperature stabilization. The resulting increase in the accuracy–time stabilization ratio makes it possible for those who conduct low-temperature experiments to improve the quality of their measurements dramatically. The introduction of a digital version of the temperature control device opens up possibilities for further automation of cryovacuum units by linking the thermal control program with other programs, for example, recording the spectra at specific temperature values.
Keywords: automation, temperature control, low temperatures, PID-controllers, IR spectroscopy, programming
Acknowledgements. These studies have been carried out with the financial support of the Ministry of Education and Science of the Republic of Kazakhstan under grant AP08855681.
References
Acknowledgements. These studies have been carried out with the financial support of the Ministry of Education and Science of the Republic of Kazakhstan under grant AP08855681.
References
-
Lee Y., Halperin W.P. Recent progress and new challenges in quantum fluids and solids. Journal of Low Temperature Physics, 2017, vol. 189, no. 1, pp. 1–14. https://doi.org/10.1007/s10909-017-1800-4
-
Jones A.T., Scheller C.P., Prance J.R., Kalyoncu Y.B., Zumbühl D.M., Haley R.P. Progress in cooling nanoelectronic devices to ultra-low temperatures. Journal of Low Temperature Physics, 2020, vol. 201, no. 5, pp. 772–802. https://doi.org/10.1007/s10909-020-02472-9
-
Debenedetti P.G., Stillinger F.H. Supercooled liquids and the glass transition. Nature, 2001, vol. 410, no. 6825, pp. 259–267. https://doi.org/10.1038/35065704
-
Cavagna A. Supercooled liquids for pedestrians. Physics Reports, 2009, vol. 476, no. 4-6, pp. 51–124. https://doi.org/10.1016/j.physrep.2009.03.003
-
Ediger M.D., Angell C.A., Nagel S.R. Supercooled liquids and glasses. The Journal of Physical Chemistry, 1996, vol. 100, no. 31, pp. 13200–13212. https://doi.org/10.1021/jp953538d
-
Hodge I.M. Enthalpy relaxation and recovery in amorphous materials. Journal of Non-Crystalline Solids, 1994, vol. 169, no. 3, pp. 211–266. https://doi.org/10.1016/0022-3093(94)90321-2
-
Yokoyama D. Molecular orientation in small-molecule organic light-emitting diodes. Journal of Materials Chemistry, 2011, vol. 21, no. 48, pp. 19187–19202. https://doi.org/10.1039/C1JM13417E
-
Galliou S., Imbaud J., Goryachev M., Bourquin R., Abbé P. Losses in high quality quartz crystal resonators at cryogenic temperatures. Applied Physics Letters, 2011, vol. 98, no. 9, pp. 091911. https://doi.org/10.1063/1.3559611
-
Buehler W.J., Gilfrich J.V., Wiley R.C. Effect of low‐temperature phase changes on the mechanical properties of alloys near composition TiNi.Journal of Applied Physics, 1963, vol. 34, no. 5, pp. 1475–1477. https://doi.org/10.1063/1.1729603
-
Öberg K.I., Garrod R.T., van Dishoeck E.F., Linnartz H. Formation rates of complex organics in UV irradiated CH3OH-rich ices. I. Experiments. Astronomy & Astrophysics, 2009, vol. 504, no. 3, pp. 891–913. http://doi.org/10.1051/0004-6361/200912559
-
Parise B., Castets A., Herbst E., Caux E., Ceccarelli C., Mukhopadhyay I., Tielens A.G.G.M. First detection of triply-deuterated methanol. Astronomy & Astrophysics, 2004, vol. 416, no. 1, pp. 159–163. http://doi.org/10.1051/0004-6361:20034490
-
Aldiyarov A., Aryutkina M., Drobyshev A., Kaikanov M., Kurnosov V. Investigation of dynamic glass transitions and structural transformations in cryovacuum condensates of ethanol. Low Temperature Physics, 2009, vol. 35, no. 4, pp. 251–255. https://doi.org/10.1063/1.3114588
-
Drobyshev A., Aldiyarov A., Zhumagaliuly D., Kurnosov V., Tokmoldin N. Thermal desorption and IR spectrometric investigation of polyamorphic and polymorphic transformations in cryovacuum condensates of water. Low Temperature Physics, 2007, vol. 33, no. 5, pp. 472–480. https://doi.org/10.1063/1.2737563
-
Aldiyarov A., Aryutkina M., Drobyshev A., Kurnosov V. IR spectroscopy of ethanol in nitrogen cryomatrices with different concentration ratios. Low Temperature Physics, 2011, vol. 37, no. 6, pp. 524–531. https://doi.org/10.1063/1.3622633
-
Drobyshev A., Aldiyarov A., Katpaeva K., Korshikov E., Kurnosov V., Sokolov D. Transformation of cryovacuum condensates of ethanol near the glass transition temperature. Low Temperature Physics, 2013, vol. 39, no. 8, pp. 714–718. https://doi.org/10.1063/1.4818634
-
Pontoppidan K.M., Fraser H.J., Dartois E., Thi W.-F., van Dishoeck E.F., Boogert A.C.A., d'Hendecourt L., Tielens A.G.G.M., Bisschop S.E. A 3-5 μm VLT spectroscopic survey of embedded young low mass stars I Structure of the CO ice. Astronomy & Astrophysics, 2003, vol. 408, no. 3, pp. 981–1007. https://doi.org/10.1051/0004-6361:20031030
-
McCarthy C., Castillo-Rogez J.C. Planetary ices attenuation properties. The Science of Solar System Ices. New York, Springer, 2013, pp. 183–225. https://doi.org/10.1007/978-1-4614-3076-6_7
-
Moore M.H., Hudson R.L. Far-infrared spectra of cosmic-type pure and mixed ices. Astronomy and Astrophysics Supplement Series, 1994, vol. 103, pp. 45–56.
-
Interstellar Dust: Proceedings of the 135th Symposium of the International Astronomical Union, Held in Santa Clara, California, July 26–30, 1988. Ed by L.J. Allamandola, A.G.G.M. Tielens. Springer Science & Business Media, 1989, XVI, 526 p. https://doi.org/10.1007/978-94-009-2462-8
-
Baragiola R.A. Water ice on outer solar system surfaces: Basic properties and radiation effects. Planetary and Space Science, 2003, vol. 51, no. 14-15, pp. 953–961. https://doi.org/10.1016/j.pss.2003.05.007
-
Ferraro J.R., Sill G., Fink U. Infrared intensity measurements of cryodeposited thin films of NH3, NH4HS, H2S, and assignments of absorption bands. Applied Spectroscopy, 1980, vol. 34, no. 5, pp. 525–533. https://doi.org/10.1366/0003702804731339
-
Warren S.G. Optical constants of carbon dioxide ice. Applied Optics, 1986, vol. 25, no. 16, pp. 2650–2674. https://doi.org/10.1364/AO.25.002650