Menu
Publications
2024
2023
2022
2021
2020
2019
2018
2017
2016
2015
2014
2013
2012
2011
2010
2009
2008
2007
2006
2005
2004
2003
2002
2001
Editor-in-Chief
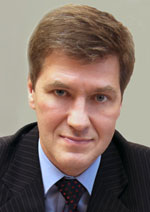
Nikiforov
Vladimir O.
D.Sc., Prof.
Partners
doi: 10.17586/2226-1494-2023-23-1-178-191
Numerical and analytical modeling of the propulsive wing and fuselage of an air taxi
Read the full article

Article in Russian
For citation:
Abstract
For citation:
Bulat P.V., Kurnukhin A.A., Prodan N.V. Numerical and analytical modeling of the propulsive wing and fuselage of an air taxi. Scientific and Technical Journal of Information Technologies, Mechanics and Optics, 2023, vol. 23, no. 1, pp. 178–191 (in Russian). doi: 10.17586/2226-1494-2023-23-1-178-191
Abstract
The problem of creating propulsive airfoils is considered. Such airfoils have a slit through which the boundary layer is sucked out. Located just behind this gap, a specially profiled section of the airfoil creates a propulsive thrust. The thrust is created due to an abrupt change in the pressure profile on the slit through which the boundary layer is sucked. In the last 15–20 years, the concept of a so-called propulsive wing with reduced or zero aerodynamic drag due to the suction of the boundary layer from its upper surface has been actively studied in the world. Such a wing makes it possible to reduce the aerodynamic drag of the aircraft by several times due to boundary layer laminarization and minimizing the velocity defect associated with viscous friction in the boundary layer, in the wake of the aircraft. The paper proposes a method for numerical modeling of airfoils for a propulsive wing constructed by solving the inverse problem of aerodynamics. The designed airfoils have a maximum construction height, an optimal combination of the lifting force coefficient Cl and the thrust coefficient CT, created by air suction from the wing surface. The developed technique correctly predicts the point of the laminar-turbulent transition, since the characteristics of the airfoils directly depend on the length of the laminar section. The layout of an aircraft built according to the scheme of a propulsive flying wing of ultra-small aspect ratio using the developed airfoils has been studied. The design of aerodynamic profiles was carried out by solving the inverse problem of aerodynamics with subsequent refinement of geometry using global optimization algorithms. Calculations were carried out using the Langtry−Menter turbulence γ-ReΘ Transition Shear Stress Transport model, in which there are relations for the intermittency criterion, makes it possible to simulate a laminar-turbulent transition. Calculations have shown that the developed airfoils make it possible to create an aircraft airframe with a maximum lift coefficient Clmax which exceeds the Clmax of a mechanized wing with a flap released during takeoff and landing. In horizontal flight, the Cl is three times larger than that of a typical wing. The wing with the developed profiles has a high propulsive efficiency due to the proximity of pressure and velocity in the thrust section of the airfoils and external flow. At the same time, the thrust surface of the propulsive wing exceeds the nozzle area or the total coverage of aircraft propellers by several times. The developed airfoils and integrated aerodynamic layout of the aircraft are well combined with the principles of building a distributed power plant, and allow you to combine immunity to increased atmospheric turbulence during vertical takeoff and landing with economical horizontal flight. Airfoils have an important advantage over traditional wing mechanization because they have no moving parts, and the increase or decrease in lift is regulated by changing the flow rate of the sucked air.
Keywords: aerodynamic profile, mathematical modeling, optimization, propulsive wing, numerical experiment, power aerodynamic
Acknowledgements. The work was carried out with the financial support of the Ministry of Science and Higher Education of the Russian Federation during the implementation of the project “Fundamentals of mechanics, control and control systems of unmanned aircraft systems with form-forming structures deeply integrated with power plants and unique properties not used today in manned aviation”, No. FEFM-2020-0001.
References
Acknowledgements. The work was carried out with the financial support of the Ministry of Science and Higher Education of the Russian Federation during the implementation of the project “Fundamentals of mechanics, control and control systems of unmanned aircraft systems with form-forming structures deeply integrated with power plants and unique properties not used today in manned aviation”, No. FEFM-2020-0001.
References
-
Felder J.L., Tong M.T., Chu J. sensitivity of mission energy consumption to turboelectric distributed propulsion design assumptions on the N3-X hybrid wing body aircraft. Proc. of the 48th AIAA/ASME/SAE/ASEE Joint Propulsion Conference and Exhibit, 2012. https://doi.org/10.2514/6.2012-3701
-
Bradley M., Droney C. Subsonic Ultra Green Aircraft Research. Phase I Final Report. NASA/CR2011-216847. 2011, 193 p.
-
Felder J.L., Kim H.D., Brown G. An examination of the effect of boundary layer ingestion on turboelectric distributed propulsion systems. Proc. of the 49th AIAA Aerospace Sciences Meeting including the New Horizons Forum and Aerospace Exposition, 2011. https://doi.org/10.2514/6.2011-300
-
Alrashed M., Nicoladis T., Pilidis P., Jafari S. Utilisation of turboelectric distribution propulsion in commercial aviation: A review on NASA’s TeDP concept. Chinese Journal of Aeronautics, 2021, vol. 34, no. 11, pp. 48–65. https://doi.org/10.1016/j.cja.2021.03.014
-
Chen Z., Zhang M., Chen Y., Sang W., Tan Z., Li D., Zhang B. Assessment on critical technologies for conceptual design of blended-wing-body civil aircraft. Chinese Journal of Aeronautics, 2019, vol. 32, no. 8, pp. 1797–1827. https://doi.org/10.1016/j.cja.2019.06.006
-
Yaros S.F., Sexstone M.G., Huebner L.D., Lamar J.E., McKinley R.E., Jr., Torres A.O., Burley C.L., Scott R.C., Small W.J. Synergistic Airframe-Propulsion Interactions and Integrations. A White Paper Prepared by the 1996-1997 Langley Aeronautics Technical Committee. NASA/TM-1998-207644, 1998, 122 p.
-
Burston M., Ranasinghe K., Gardi A., Parezanovic V., Ajaj R., Sabatini R. Design principles and digital control of advanced distributed propulsion systems. Energy, 2022, vol. 241, pp. 122788. https://doi.org/10.1016/j.energy.2021.122788
-
Richards E.J., WalkerW.S., GreeningJ. R. Tests of a Griffith Aerofoil in the 13 ft x 9 ft Wind Tunnel. Part 1, Part 2, Part 3, Part 4. ARC R&M-2148 ARC-7464 ARC-7561 ARC-8054 ARC-8055. 1944.
-
Goldschmied F. Fuselage self-propulsion by static-pressure thrust: Wind-tunnel verification. Proc. of the Aircraft Design, Systems and Operations Meeting, 1987. https://doi.org/10.2514/6.1987-2935
-
Goldschmied F. Airfoil static-pressure thrust: flight test verification. Proc. of the Aircraft Design, Systems and Operations Conference, 1990. https://doi.org/10.2514/6.1990-3286
-
Goldschmied F. Thick-wing spanloader all-freighter: design concept for tomorrow’s air cargo. Proc. of the Aircraft Design, Systems and Operations Conference, 1990. https://doi.org/10.2514/6.1990-3198
-
Cella U., Quagliarella D., Donelli R. Imperatore B. Design and test of the UW-5006 transonic natural-laminar-flow wing. Journal of Aircraft, 2010, vol. 47, no. 3, pp. 783–795. https://doi.org/10.2514/1.40932
-
Bulat P.V., Kurnukhin A.A., Prodan N.V. Numerical simulation of propulsive aerodynamic profiles. Scientific and Technical Journal of Information Technologies, Mechanics and Optics, 2022, vol. 22, no. 5, pp. 1007–1015. (in Russian). https://doi.org/10.17586/2226-1494-2022-22-5-1007-1015
-
Bulat P.V., Dudnikov S.Iu., Kuznetcov P.N. Fundamental Aerodynamics of the Unmanned Aerial Vehicles. Tutorial. Moscow, “Sputnik+” Publ., 2021, 273 p. (in Russian)
-
Tamaki T., Nagano S. Effects of inlet distortions on a multi-stage compressor. Proc. of the 4th International Symposium on Air Breathing Engines, 1979. https://doi.org/10.2514/6.1979-7003
-
Sandercock D.M., Sanger N.L. Some observations of the effects of radial distortions on performance of a transonic rotating blade row. NASA TN D-7824, 1974, 48 p.
-
Hancock J.P. Test of a high efficiency transverse fan. Proc. of the 16th Joint Propulsion Conference, 1980. https://doi.org/10.2514/6.1980-1243
-
Harloff G.J., Wilson D.R. Cross-flow propulsion fan experimental development and finite-element modeling. Journal of Aircraft, 1981, vol. 18, no. 4, pp. 310–317. https://doi.org/10.2514/3.57494
-
Dygert R.K., Dang T.Q. Experimental investigation of embedded cross-flow fan for airfoil propulsion/circulation control. Proc. of the 45th AIAA Aerospace Sciences Meeting and Exhibit, 2007. https://doi.org/10.2514/6.2007-368
-
Gologan C., Mores S., Steiner H., Seitz A. Potential of the cross-flow fan for powered-lift regional aircraft applications. Proc. of the 9th AIAA Aviation Technology, Integration, and Operations Conference (ATIO), 2009. https://doi.org/10.2514/6.2009-7098
-
Langel C.M., Chow R., Van Dam C.P., Maniaci D.C., Erhmann R.S., White E.B. A computational approach to simulating the effects of realistic surface roughness on boundary layer transition. Proc. of the 52nd Aerospace Sciences Meeting, 2014. https://doi.org/10.2514/6.2014-0234
-
Doll U., Migliorini M., Baikie J., Zachos K.P., Röhle I., Melnikov S., Steinbock J., Dues M., Kapulla R., MacManus D.G., Lawson N.J. Non-intrusive flow diagnostics for unsteady inlet flow distortion measurements in novel aircraft architectures. Progress in Aerospace Sciences, 2022, vol. 130, pp. 100810. https://doi.org/10.1016/j.paerosci.2022.100810
-
Dang T.Q., Bushnell P.P. Aerodynamics of cross-flow fans and their application to aircraft propulsion and flow control. Progress in Aerospace Sciences, 2009, vol. 45, no. 1-3, pp. 1–29. https://doi.org/10.1016/j.paerosci.2008.10.002
-
Karpuk S.V., Kazarin P., Gudmundsson S., Golubev V.V. Preliminary feasibility study of a multi-purpose aircraft concept with a leading-edge embedded cross-flow fan. Proc. of the 2018 AIAA Aerospace Sciences Meeting, 2018. https://doi.org/10.2514/6.2018-1744
-
Kulkarni A.R., La Rocca G., Veldhuis L.L.M., Eitelberg G. Sub-scale flight test model design: Developments, challenges and opportunities. Progress in Aerospace Sciences, 2022, vol. 130, pp. 100798. https://doi.org/10.1016/j.paerosci.2021.100798
-
Perry A.T., Ansell P.J., Kerho M., Ananda G., D’Urso S. Design, analysis, and evaluation of a propulsive wing concept. Proc. of the 34th AIAA Applied Aerodynamics Conference, 2016. https://doi.org/10.2514/6.2016-4178
-
Prodan N.V., Kurnukhin A.A. Application of mathematical optimization methods for designing airfoil considering viscosity. Russian Aeronautics, 2021, vol. 64, no. 4, pp. 670–677. https://doi.org/10.3103/S1068799821040115
-
Kramer B., Ansell Ph., D’Urso S., Ananda G., Perry A. Design, Analysis, and Evaluation of a Novel Propulsive Wing Concept. LEARN Phase I. Final Report. Contract Number NNX15AE39A. June 30th, 2016, 80 p.
-
Bulat P.V., Prodan N.V., Kurnukhin A.A. On the influence of the laminar-turbulent transition in the numerical modeling of the wing airfoil. Russian Aeronautics, 2021, vol. 64, no. 3, pp. 455–465. https://doi.org/10.3103/S1068799821030120
-
Abzalilov D.F., Mardanov R.F. Calculation and optimization of aerodynamic characteristics of airfoils with jet blowing in the presence of vortex in the flow. Russian Aeronautics, 2016, vol. 59, no. 3, pp. 358–363. https://doi.org/10.3103/S1068799816030107
-
Drela M. XFOIL: An analysis and design system for low Reynolds number airfoils. Lecture Notes in Engineering, 1989, vol. 54, pp. 1–12. https://doi.org/10.1007/978-3-642-84010-4_1
-
Langtry R.B., Menter F.R. Transition modeling for general CFD applications in aeronautics. Proc. of the 43rd AIAA Aerospace Sciences Meeting and Exhibit, 2005. https://doi.org/10.2514/6.2005-522
-
Malan P., Suluksna K., Juntasaro E. Calibrating the γ-Reθ transition model for commercial CFD. Proc. of the 47th AIAA Aerospace Sciences Meeting including The New Horizons Forum and Aerospace Exposition, 2009. https://doi.org/10.2514/6.2009-1142
-
Liu K., Wang Y., Song W.-P., Han Z.-H. A two-equation local-correlation-based laminar-turbulent transition modeling scheme for external aerodynamics. Aerospace Science and Technology, 2020, vol. 106, pp. 106128. https://doi.org/10.1016/j.ast.2020.106128
-
Solomatin R.S., Semenov I.V., Men'shov I.S. Towards calculating turbulent flows with the Spalart-Allmaras model by using the LU-SGS-GMRES algorithm. Keldysh Institute Preprints, 2018, no. 119, pp. 1–30. (in Russian)
-
Dudnikov S.Y., Kuznetsov P.N., Mel’nikova A.I., Vokin L.O. Simulation of flows at low Reynolds numbers as applied to the design of aerodynamic surfaces for unmanned aerial vehicles. Russian Aeronautics, 2021, vol. 64, no. 4, pp. 620–629. https://doi.org/10.3103/S1068799821040061
-
Bulat P.V., Prodan N.V., Dudnikov S.YU., Kurnukhin A.A. Investigation of the characteristics of airfoils with air suction from the upper surface and a given pressure distribution. Izvestiya vysshikh uchebnykh zavedenii, 2022, no. 3. (in Russian)