Menu
Publications
2025
2024
2023
2022
2021
2020
2019
2018
2017
2016
2015
2014
2013
2012
2011
2010
2009
2008
2007
2006
2005
2004
2003
2002
2001
Editor-in-Chief
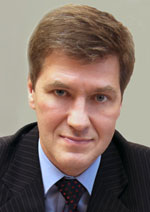
Nikiforov
Vladimir O.
D.Sc., Prof.
Partners
doi: 10.17586/2226-1494-2023-23-2-218-226
Modern approaches to the application of mathematical modeling methods in biomedical research
Read the full article

Article in Russian
For citation:
Abstract
For citation:
Krasnikov I.V., Seteikin A.Yu., Roth B. Modern approaches to the application of mathematical modeling methods in biomedical research. Scientific and Technical Journal of Information Technologies, Mechanics and Optics, 2023, vol. 23, no. 2, pp. 218–226 (in Russian). doi: 10.17586/2226-1494-2023-23-2-218-226
Abstract
This paper presents a brief overview of the main approaches to mathematical modeling of the interaction of optical radiation with biological tissues. In the case of light propagation in tissue, the Monte Carlo method is an approximation of the solution of the radiation transfer equation. This is done by sampling the set of all possible trajectories of light quanta (photon packets) as they pass through the tissue. Such a stochastic model makes it possible to simulate the propagation of light in a turbid (scattering) medium. The main types of interaction between photons and tissue are considered: scattering, absorption, and reflection/refraction at the boundary of the medium. The algorithm of the method is based on the statistical approximation of the estimated parameters instead of using non-linear functional transformations. Efficient methods for modeling the problem of Raman spectroscopy in turbid media are shown, taking into account the parameters of the detector and the sample size. Two fundamental approaches to the numerical simulation of Raman scattering are considered. Based on data from open literary sources, a variant of modeling Raman scattering in normal multilayer human skin in the near infrared wavelength range is shown. The Raman spectra of ex vivo normal skin tissue sections are presented to quantify various intrinsic micro spectral properties of different skin layers. The reconstructed Raman spectrum of the skin is compared with clinically measured skin spectra in vivo. The overall good agreement between the simulated process and experimental data is shown. The possibility of using the sequential Monte Carlo method for data processing in correlation wide-field optical coherence tomography for the study of biological objects is shown.
Keywords: optical radiation, absorption, scattering, modeling, biological tissues, Monte Carlo method
References
References
-
Tuchin V.V. Biological Tissue Optics. Light Scattering Methods in Medical Diagnostics. Moscow, Fizmatlit Publ., 2012, 812 p. (in Russian)
-
Arridge S.R. Optical tomography: forward and inverse problems. Inverse Problems, 2009, vol. 25, no. 12, pp. 123010. https://doi.org/10.1088/0266-5611/25/12/123010
-
Bassi A., D'Andrea C., Valentini G., Cubeddu R., Arridge S.R. Temporal propagation of spatial information in turbid media.Optics Letters, 2008, vol. 33, no. 23, pp. 2836–2838. https://doi.org/10.1364/OL.33.002836
-
Meglinski I., Doronin A.V. Monte Carlo modeling of photon migration for the needs of biomedical optics and biophotonics.Advanced Biophotonics,2012, pp. 1–72.
-
Fischer D.G., Prahl S.A., Duncan D.D. Monte Carlo modeling of spatial coherence: free-space diffraction.Journal of the Optical Society of America A, 2008, vol. 25, no. 10,pp. 2571–2581. https://doi.org/10.1364/JOSAA.25.002571
-
Jacques S. Monte Carlo modeling of light transport in tissue.Optical-thermal response of laser-irradiated tissue.Springer Netherlands, 2010, pp. 109–144. https://doi.org/10.1007/978-90-481-8831-4_5
-
Flock S.T., Patterson M.S., Wilson B.C., Wyman D.R. Monte Carlo modeling of light propagation in highly scattering tissues. I. Model predictions and comparison with diffusion theory.IEEE Transactions on Biomedical Engineering, 1989,vol. 36, no. 12,pp. 1162–1168. https://doi.org/10.1109/tbme.1989.1173624
-
Flock S.T., Wilson B.C., Patterson M.S. Monte Carlo modeling of light propagation in highly scattering tissues. II. Comparison with measurements in phantoms.IEEE Transactions on Biomedical Engineering, 1989, vol. 36, no. 12, pp. 1169–1173. https://doi.org/10.1109/10.42107
-
Alerstam E., Lo W., Han T., Rose J., Andersson-Engels S., Lilge L. Next-generation acceleration and code optimization for light transport in turbid media using GPUs.Biomedical Optics Express, 2010, vol. 1, no. 2, pp. 658–675. https://doi.org/10.1364/boe.1.000658
-
Li P., Liu C., Li X., He H., Ma H. GPU acceleration of Monte Carlo simulations for polarized photon scattering in anisotropic turbid media.Applied Optics, 2016, vol. 55, no. 27,pp. 7468–7476. https://doi.org/10.1364/ao.55.007468
-
Doronin A., Meglinski I. Peer-to-peer Monte Carlo simulation of photon migration in topical applications of biomedical optics.Journal of Biomedical Optics, 2012, vol. 17, no. 9, pp. 090504. https://doi.org/10.1117/1.jbo.17.9.090504
-
Korhonen V.O., Myllyla T.S., Kirillin M.Yu., Popov A.P., Bykov A.V., Gorshkov A.V., Sergeeva E.A., Kinnunen M., Kiviniemi V. Light propagation in NIR spectroscopy of the human brain.IEEE Journal of Selected Topics in Quantum Electronics, 2014, vol. 20, no. 2,pp. 7100310. https://doi.org/10.1109/jstqe.2013.2279313
-
Wang L.V., Wu H.-I. Biomedical Optics: Principles and Imaging. Hoboken, NJ, USA, Wiley, 2009, 376 p.
-
Yun S.H., Kwok S.J. Light in diagnosis, therapy and surgery.Nature Biomedical Engineering, 2017, vol. 1,no. 1, pp. 0008. https://doi.org/10.1038/s41551-016-0008
-
TianJ., Liu K., Lu Y., Qin C., Yang X., Zhu S., Han D., Feng J., Ma X., Chang Z. Evaluation of the simplified spherical harmonics approximation in bioluminescence tomography through heterogeneous mouse mode. Optics Express, 2010, vol. 18, no. 20, pp. 20988–21002. https://doi.org/10.1364/oe.18.020988
-
Jacques S.L., Wang L. Animated simulation of light transport in tissues. Proceedings of SPIE, 1994, vol. 2134, pp. 2134A. https://doi.org/10.1117/12.182939
-
Periyasamy V., Pramanik M. Advances in Monte Carlo simulation for light propagation in tissue. IEEE Reviews in Biomedical Engineering, 2017, vol. 10, pp. 122–135. https://doi.org/10.1109/rbme.2017.2739801
-
Gandjbakhche A.H., Weiss G.H., Bonner R.F., Nossal R. Photon path-length distributions for transmission through optically turbid slabs. Physical Review E, 1993, vol. 48, no. 2, pp. 810–818. https://doi.org/10.1103/physreve.48.810
-
Li X., Ma L. Scaling law for photon transmission through optically turbid slabs based on random walk theory. Applied Sciences, 2012, vol. 2, no. 1, pp. 160–165. https://doi.org/10.3390/app2010160
-
Sun X., Li X., Ma L. A closed-form method for calculating the angular distribution of multiply scattered photons through isotropic turbid slabs. Optics Express, 2011, vol. 19, no. 24, pp. 23932–23937. https://doi.org/10.1364/oe.19.023932
-
Optical-Thermal Response of Laser-Irradiated Tissue. V. 2. Ed. by A.J. Welch, M.J. Van Gemert. Springer Science+Business Media B.V., 2011, 958 p. https://doi.org/10.1007/978-90-481-8831-4
-
Lin Y., Northrop W.F., Li X. Markov chain solution of photon multiple scattering through turbid slabs. Optics Express, 2016, vol. 24, no. 23, pp. 26942–26947. https://doi.org/10.1364/OE.24.026942
-
Xu F., Davis A.B., Sanghavi S.V., Martonchik J.V., Diner D. Linearization of Markov chain formalism for vector radiative transfer in a plane-parallel atmosphere/surface system. Applied Optics, 2012, vol. 51, no. 16, pp. 3491–3507. https://doi.org/10.1364/ao.51.003491
-
Zhu C., Liu Q. Review of Monte Carlo modeling of light transport in tissues. Journal of Biomedical Optics, 2013, vol. 18, no. 5, pp. 50902. https://doi.org/10.1117/1.jbo.18.5.050902
-
Drexler W., Liu M., Kumar A., Kamali T., Unterhuber A., Leitgeb R.A. Optical coherence tomography today: speed, contrast, and multimodality. Journal of Biomedical Optics, 2014, vol. 19, no. 7, pp. 71412. https://doi.org/10.1117/1.JBO.19.7.071412
-
Wieser W., Draxinger W., Klein T., Karpf S., Pfeiffer T., Huber R. High definition live 3D-OCT in vivo: design and evaluation of a 4D OCT engine with 1 GVoxel/s. Biomedical Optics Express, 2014, vol. 5, no. 9, pp. 2963–2977. https://doi.org/10.1364/BOE.5.002963
-
Kim J., Brown W., Maher J.R., Levinson H., Wax A. Functional optical coherence tomography: principles and progress. Physics in Medicine and Biology, 2015, vol. 60, no. 10, pp. 211–237. https://doi.org/10.1088/0031-9155/60/10/r211
-
Thrane L. Optical Coherence Tomography: Modeling and applications. Risø National Laboratory, 2001, 76 p.
-
Smithies D.J., Lindmo T., Chen Z., Nelson J.S., Milner T.E. Signal attenuation and localization in optical coherence tomography studied by Monte Carlo simulation. Physics in Medicine and Biology, 1998, vol. 43, no. 10, pp. 3025–3044. https://doi.org/10.1088/0031-9155/43/10/024
-
Varkentin A., Otte M., Meinhardt-Wollweber M., Rahlves M., Mazurenka M., Morgner U., Roth B. Simple model to simulate OCT-depth signal in weakly and strongly scattering homogeneous media. Journal of Optics, 2016, vol. 18, no. 12, pp. 125302. https://doi.org/10.1088/2040-8978/18/12/125302
-
Kirillin M., Farhat G., Sergeeva E., Kolios M., Vitkin A. Speckle statistics in OCT images: Monte Carlo simulations and experimental studies. Optics Letters, 2014, vol. 39, no. 12, pp. 3472–3475. https://doi.org/10.1364/ol.39.003472
-
Kirillin M., Agrba P., Kamensky V. Mechanical compression in cross-polarization OCT imaging of skin: In vivo study and Monte Carlo simulation. Photonics & Lasers in Medicine, 2014, vol. 3, no. 4, pp. 363–372. https://doi.org/10.1515/plm-2014-0015
-
Shih W.-C., Bechtel K.L., Feld M.S. Intrinsic Raman spectroscopy for quantitative biological spectroscopy. Part I: theory and simulations. Optics Express, 2008, vol. 16, no. 17, pp. 12726–12736. https://doi.org/10.1364/OE.16.012726
-
Krasnikov I., Suhr C., Seteikin A., Roth B., Meinhardt-Wollweber M. Two efficient approaches for modeling of Raman scattering in homogeneous turbid media. Journal of the Optical Society of America A, 2016, vol. 33, no. 3, pp. 426–433. https://doi.org/10.1364/JOSAA.33.000426
-
Everall N., Hahn T., Matousek P., Parker A.W., Towrie M. Photon migration in Raman spectroscopy. Applied Spectroscopy, 2004, vol. 58, pp. 591–597. https://doi.org/10.1366/000370204774103426
-
Krasnikov I., Seteikin A., Kniggendorf A.-K., Meinhardt-Wollweber M., Roth B. Simulation of Raman scattering including detector parameters and sampling volume. Journal of the Optical Society of America A, 2017, vol. 34, no. 12, pp. 2138–2144. https://doi.org/10.1364/JOSAA.34.002138
-
Zherebtsov E., Dremin V., Popov A., Doronin A., Kurakina D., Kirillin M., Meglinski I., Bykov A. Hyperspectral imaging of human skin aided by artificial neural networks. Biomedical Optics Express, 2019, vol. 10, no. 7, pp. 3545–3559. https://doi.org/10.1364/BOE.10.003545
-
Reble C., Gersonde I., Andree S., Eichler H.J., Helfmann J. Quantitative Raman spectroscopy in turbid media. Journal of Biomedical Optics, 2010, vol. 15, no. 3, pp. 037016. https://doi.org/10.1117/1.3456370
-
Handbook of Optical Biomedical Diagnostics. Vol. PM107. Ed. by V. Tuchin. Washington, SPIE Press, 2002, 1110 p.
-
Wang S., Zhao J., Lui H., He Q., Bai J., Zeng H. Monte Carlo simulation of in vivo Raman spectral measurements of human skin with a multi-layered tissue optical model. Journal of Biophotonics, 2014, vol. 7, no. 9, pp. 703–712. https://doi.org/10.1002/jbio.201300045
-
Zeng H., MacAulay C., McLean D.I., Palcic B. Reconstruction of in vivo skin autofluorescence spectrum from microscopic properties by Monte Carlo simulation. Journal of Photochemistry and Photobiology B: Biology, 1997, vol. 38, no. 2-3, pp. 234–240. https://doi.org/10.1016/s1011-1344(96)00008-5
-
Chen R., Huang Z., Lui H., Hamzavi I., McLean D.I., Xie S., Zeng H. Monte Carlo simulation of cutaneous reflectance and fluorescence measurements – The effect of melanin contents and localization. Journal of Photochemistry and Photobiology B: Biology, 2007, vol. 86, no. 3, pp. 219–226. https://doi.org/10.1016/j.jphotobiol.2006.11.001
-
Wang S., Zhao J., Lui H., He Q., Zeng H. Monte Carlo simulation of near infrared autofluorescence measurements of in vivo skin. Journal of Photochemistry and Photobiology B: Biology, 2011, vol. 105, no. 3, pp. 183–189. https://doi.org/10.1016/j.jphotobiol.2011.08.008
-
Wang S., Zhao J., Lui H., He Q., Zeng H. A modular Raman microspectroscopy system for biological tissue analysis. Spectroscopy, 2010, vol. 24, no. 6, pp. 577–583. https://doi.org/10.1155/2010/592315