Menu
Publications
2024
2023
2022
2021
2020
2019
2018
2017
2016
2015
2014
2013
2012
2011
2010
2009
2008
2007
2006
2005
2004
2003
2002
2001
Editor-in-Chief
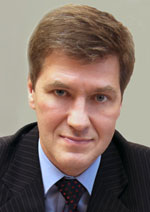
Nikiforov
Vladimir O.
D.Sc., Prof.
Partners
doi: 10.17586/2226-1494-2023-23-3-659-667
Method of type-C liquified natural gas tank modeling based on volume optimization for future “milk-run” exploitation
Read the full article

Article in English
For citation:
Abstract
For citation:
Ivanov L.V., Baranov A.Yu., Novitskaya A.V. Method of type-C liquified natural gas tank modeling based on volume optimization for future “milk-run” exploitation. Scientific and Technical Journal of Information Technologies, Mechanics and Optics, 2023, vol. 23, no. 3, pp. 659–667. doi: 10.17586/2226-1494-2023-23-3-659-667
Abstract
Common practice methods of tank design for transportation of liquefied natural gas don’t take into account the specifics of the gas carriers operation under the condition of partial filling of cryogenic tanks. A new method for designing of type-C tank is proposed. Method is based on solving the problem of increasing the volume of transported liquefied natural gas by small-scale inland carriers. The method is based on usage of a number of limiting parameters: minimal allowable ventless operation time, allowable values of the ship’s draft, and the actual duration of voyages between neighboring consumers. The method allows optimizing type, shape, wall thickness, and heat insulation thickness of cryogenic tank. The proposed method is aimed at enlargement of usage of the ship’s hull dimensions. This is achieved by changing the diameter, the distance between centers of the bi-lobe tank, the thickness of the insulation, and the maximum allowable working pressure. An increase in the volume of the tank is achieved by coordination such parameters as the maximum allowable draft of the vessel, the minimum time of ventless storage, and the time of ventless operation under partial filling conditions. The calculation of the ventless operation time is determined by the operating conditions of type-C tanks. The calculation of the heat ingress into the tank takes into account the contact area of liquefied gas and its vapors with the metal wall of the tank. The calculations do not take into account the assumption of thermal equilibrium between the liquid and vapor fractions, which leads to the need to take into account heat transfer from vapor to liquid. The implementation of the method is shown on the example of the modeling of the two-way river-sea type vessel. It is shown that optimization of tank parameters in accordance with proposed criteria can lead to an increase in the volume of transported natural gas by more than 4 %. The method can be used in the development of new and modernization of existing vessel projects to transportation of liquefied natural gas operating in water basins of Lena and Yenisei rivers in the East Siberian region. The described method can also be used in the design of road and rail tanks as well as small- scale bullet tanks for liquefied natural gas.
Keywords: cryogenic tank design, type-C tank, partial filling, tank optimization, inland carrier, small-scale tanker, liquiefied natural gas, ventless operation
References
References
-
Lee S., Seo Y., Lee J., Chang D. Economic evaluation of pressurized LNG supply chain. Journal of Natural Gas Science and Engineering, 2016, vol. 33, pp. 405–418. https://doi.org/10.1016/j.jngse.2016.05.039
-
Knizhnikov A.Yu., Klimentiev A.Yu. Perspective and opportunities of usage of LNG in arctic regions of Russia. Conference WWF View Russia, 2018, Moscow.
-
Ivanov L.V., Baranov A.Yu. Analysis of technical and logistic solutions used in the inland transportation by small-scale LNG carriers.Gas Industry, 2021, no. 4(815), pp. 80–86. (in Russian)
-
Budiyanto M.A., Pamitran A.S., Yusman T. Optimization of the route of distribution of LNG using small scale LNG carrier: a case study of a gas power plant in the Sumatra region, Indonesia. International Journal of Energy Economics and Policy, 2019, vol. 9, no 6, pp. 179–187. https://doi.org/10.32479/ijeep.8103
-
Budiyanto M.A., Riadi A., Sumanta Buana I.G.N., Kurnita G. Study on the LNG distribution to mobile power plants utilizing small-scale LNG carriers. Heliyon, 2020, vol. 6, no. 7, pp. e04538 https://doi.org/10.1016/j.heliyon.2020.e04538
-
Strantzali E., Aravossis K., Livanos G.A., Nikoloudis Ch. “A decision support approach for evaluating liquefied natural gas supply options: Implementation on Greek case study”. Journal of Cleaner Production, 2019, vol. 222, pp. 414–423. https://doi.org/10.1016/j.jclepro.2019.03.031
-
Kalajdžić M., Bobić D. Unconventional inland LNG carrier design for the river Danube. Proc. of the 23th Symposium on Theory and Practice of Shipbuilding SORTA, 2018, pp. 426–443.
-
Huerta F., Vesovic V. A realistic vapour phase heat transfer model for the weathering of LNG stored in large tanks. Energy, 2019, vol. 174, pp. 280–291. https://doi.org/10.1016/j.energy.2019.02.174
-
Wang Y., Yun Y., Chen M., Lim Y. Numerical prediction research on the evaporation rate of liquid cargo tank for LNG Filling Vessel Based on Ansys. Proc. of the 5th International Conference on Measurement, Instrumentation and Automation (ICMIA 2016), 2016. https://doi.org/10.2991/icmia-16.2016.96
-
Lee D.-H., Cha S.-J., Kim J.-D., Kim J.-H., Kim S.-K., Lee J.-M. Practical prediction of the boil-off rate of independent-type storage tanks. Journal of Marine Science and Engineering, 2021, vol. 9, no. 1, pp. 36. https://doi.org/10.3390/jmse9010036
-
Ferrín J.L., Pérez-Pérez L.J. Numerical simulation of natural convection and boil-off in a small size pressurized LNG storage tank. Computers and Chemical Engineering, 2020, vol. 138, pp. 106840. https://doi.org/10.1016/j.compchemeng.2020.106840
-
Jo Y., Shin K., Hwang S. Development of dynamic simulation model of LNG tank and its operational strategy. Energy, 2021, vol. 223, pp. 120060. https://doi.org/10.1016/j.energy.2021.120060
-
Ivanov L.V., Baranov A.Yu., Malysheva T.A., Andreev A.M. Selection of cryogenic tanks for modernization of small-scale LNG carriers. Journal of International Academy of Refrigeration, 2021, no. 1, pp. 40–45. (in Russian). https://doi.org/10.17586/1606-4313-2021-20-1-40-45
-
Salarkia M., Golabi S., Amirsalari B. Optimum design of liquified natural gas Bi-lobe tanks using finite element, genetic algorithm and neural network. Journal of Applied and Computational Mechanics, 2020, vol. 6, no. 4, pp. 862–877. https://doi.org/10.22055/JACM.2019.14801
-
Sparks L.L. Thermal conductivity of polyurethane foam from 95 to 340 K. National Bureau of Standards, Boulder, Colorado, USA, 1982. Available at: https://nvlpubs.nist.gov/nistpubs/Legacy/IR/nbsir82-1664.pdf (accessed: 10.12.2021).
-
Arkharov A.M., Arkharov I.A., Belyakov V.P. et. al. Cryogenic Systems. In 2 vol. V. 2. Fundamentals of Designing Devices, Installations and Systems.Moscow, Mashinostroenie Publ., 1999, 720 p. (in Russian)