Menu
Publications
2024
2023
2022
2021
2020
2019
2018
2017
2016
2015
2014
2013
2012
2011
2010
2009
2008
2007
2006
2005
2004
2003
2002
2001
Editor-in-Chief
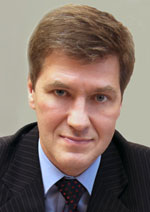
Nikiforov
Vladimir O.
D.Sc., Prof.
Partners
doi: 10.17586/2226-1494-2023-23-4-685-695
Numerical study of the effect of methemoglobin concentration in the blood on the absorption of light by human skin.
Read the full article

Article in Russian
For citation:
Abstract
For citation:
Belikov A.V., Chuchin V.Yu. Numerical study of the effect of methemoglobin concentration in the blood on the absorption of light by human skin. Scientific and Technical Journal of Information Technologies, Mechanics and Optics, 2023, vol. 23, no. 4, pp. 685–695 (in Russian). doi: 10.17586/2226-1494-2023-23-4-685-695
Abstract
Lasers are widely used in dermatology to remove telangiectasias. Increasing the efficiency of sclerosis of deep-lying and large telangiectasias with laser radiation is possible by changing the optical transmission of the skin when it is heated and converting the hemoglobin of the blood contained in it into methemoglobin. The influence of the concentration of methemoglobin in the blood on the absorption of light in human skin is poorly understood, which determines the relevance of this study in the context of finding ways to improve the efficiency of laser removal of telangiectasias. Seven-layer optical models of human skin without telangiectasia and with it for numerical simulation were developed. The extinction coefficients and the degree of change in the optical transmission of whole blood and skin layers were calculated in the range of wavelength from 400 to 1600 nm for skin model without and with arteriolar and venular telangiectasias at various concentrations of methemoglobin in the blood. Based on the analysis of these data, the wavelengths with the biggest change in the optical transmission of whole blood and skin layers occurred during the transformation of hemoglobin to methemoglobin were selected. At the selected wavelengths, the Monte Carlo method was used in optical modelling to get the distribution of the absorbed optical power in each layer of the skin model without and with telangiectasia at various concentrations of methemoglobin. It has been shown that the spectra of extinction coefficients for arteriolar and venular telangiectasias do not differ significantly. During the transformation of hemoglobin to methemoglobin, the largest decrease in the degree of change in the optical transmission of whole blood occurs at wavelengths of 629 nm and 1105 nm, and the largest increase occurs at wavelengths of 447 nm and 578 nm. The part of absorbed optical power in the layer of superficial vascular plexus without and with telangiectasia at wavelengths of 629 nm and 1105 nm increases, and at wavelengths of 441 nm and 574 nm it decreases with a growth of the methemoglobin concentration from 0 to 100 % in the skin model. At the same time, in the layer of deep vascular plexus the value of part of absorbed optical power increases at wavelengths of 441 nm, 574 nm and 1105 nm, but at a wavelength of 629 nm first increases with a growth of the methemoglobin concentration up to 25 %, and then decreases, but to values exceeding the value of part of absorbed optical power without methemoglobin. The change in optical transmission associated with the replacement of blood hemoglobin with methemoglobin is more pronounced for the superficial vascular plexus layer, which is associated with high blood content in it and a limited contribution of the overlying skin layers to the deformation of the spectrum of light incident on this layer. In skin with telangiectasia, a change in the concentration of methemoglobin changes the proportion of absorbed optical power by a greater amount than in skin without telangiectasia, which can be associated with an increase in the volume concentration of blood in skin layers with telangiectasia and an increase in their thickness. The obtained results can be applied in the development of laser systems and technologies for the treatment of skin diseases, including laser sclerosis of telangiectasias.
Keywords: absorption coefficient, scattering coefficient, skin, vessel, telangiectasias, skin optical model, laser sclerosing
References
References
- Belikov A., Khramov V. Perspective for the development of innovative research areas in the field of laser systems and biomedical optical technologies. Scientific and Technical Journal of Information Technologies, Mechanics and Optics, 2010, no. 5(69), pp. 110–114. (in Russian)
- Goldman M.P., Weiss R.A. Sclerotherapy E-Book: Treatment of Varicose and Telangiectatic Leg Veins (Expert Consult). Elsevier Health Sciences, 2016, 455 p.
- Livandovskiĭ Iu.A., Pavlova O.Iu. Telangiectasia. Klinicheskaya Dermatologiya i Venerologiya, 2010, vol. 8, no. 5, pp. 6–15. (in Russian)
- Karsai S., Roos S., Raulin C. Treatment of facial telangiectasia using a dual‐wavelength laser system (595 and 1,064 nm): a randomized controlled trial with blinded response evaluation. Dermatologic Surgery, 2008, vol. 34, no. 5, pp. 702–708. https://doi.org/10.1111/j.1524-4725.2008.34131.x
- Sadick N., Sorhaindo L. Laser treatment of telangiectasias and reticular veins. The Vein Book. Academic Press, 2007, pp. 157–166. https://doi.org/10.1016/B978-012369515-4/50019-3
- Ross E.V., Domankevitz Y. Laser treatment of leg veins: physical mechanisms and theoretical considerations. Lasers in Surgery and Medicine, 2005, vol. 36, no. 2, pp. 105–116. https://doi.org/10.1002/lsm.20141
- Nachabé R., Evers D.J., Hendriks B.H.W., Lucassen G.W., Van der Voort M., Wesseling J., Ruers T.J.M. Effect of bile absorption coefficients on the estimation of liver tissue optical properties and related implications in discriminating healthy and tumorous samples. Biomedical Optics Express, 2011, vol. 2, no. 3, pp. 600–614. https://doi.org/10.1364/boe.2.000600
- Barton J.K., Frangineas G., Pummer H., Black J.F. Cooperative phenomena in two‐pulse, two‐color laser photocoagulation of cutaneous blood vessels. Photochemistry and Photobiology, 2001, vol. 73, no. 6. pp. 642–650. https://doi.org/10.1562/0031-8655(2001)0730642cpitpt2.0.co2
- Chan E.K., Sorg B., Protsenko D., O’Neil M., Motamedi M., Welch A.J. Effects of compression on soft tissue optical properties. IEEE Journal of Selected Topics in Quantum Electronics, 1996, vol. 2, no. 4, pp. 943–950. https://doi.org/10.1109/2944.577320
- Tuchin V.V. Tissue Optics: Light Scattering Methods and Instruments for Medical Diagnosis. SPIE Press, 2015, 988 p.
- Drezek R., Dunn A., Richards-Kortum R. Light scattering from cells: finite-difference time-domain simulations and goniometric measurements. Applied Optics, 1999, vol. 38, no. 16, pp. 3651–3661. https://doi.org/10.1364/ao.38.003651
- Tuchin V.V. Optical clearing of tissues and blood using the immersion method. Journal of Physics D: Applied Physics, 2005, vol. 38, no. 15, pp. 2497–2518. https://doi.org/10.1088/0022-3727/38/15/001
- Sterenborg H.J., Van der Leun J.C. Change in epidermal transmission due to UV-induced hyperplasia in hairless mice: a first approximation of the action spectrum. Photo-dermatology, 1988, vol. 5, no. 2, pp. 71–82.
- Yaroslavsky A.N., Schulze P.C., Yaroslavsky I.V., Schober R., Ulrich F., Schwarzmaier H.-J. Optical properties of selected native and coagulated human brain tissues in vitro in the visible and near infrared spectral range. Physics in Medicine & Biology, 2002, vol. 47, no. 12, pp. 2059–2073. https://doi.org/10.1088/0031-9155/47/12/305
- Maier J.S., Walker S.A., Fantini S., Franceschini M.A., Gratton E. Possible correlation between blood glucose concentration and the reduced scattering coefficient of tissues in the near infrared. Optics Letters, 1994, vol. 19, no. 24, pp. 2062–2064. https://doi.org/10.1364/ol.19.002062
- Wen X., Mao Z., Han Z., Tuchin V.V., Zhu D. In vivo skin optical clearing by glycerol solutions: mechanism. Journal of Biophotonics, 2010, vol. 3, no. 1‐2, pp. 44–52. https://doi.org/10.1002/jbio.200910080
- Baranov V.Y., Chekhov D.I., Leonov A.G., Leonov P.G., Ryaboshapka O.M., Semenov S.Y., Tatsis G.P. Heat-induced changes in optical properties of human whole blood in vitro. Proceedings of SPIE, 1999, vol. 3599, pp. 180–187. https://doi.org/10.1117/12.348378
- Seto Y., Kataoka M., Tsuge K. Stability of blood carbon monoxide and hemoglobins during heating. Forensic Science International, 2001, vol. 121, no. 1-2, pp. 144–150. https://doi.org/10.1016/s0379-0738(01)00465-0
- Wilcox G.L., Giesler Jr G.J. An instrument using a multiple layer Peltier device to change skin temperature rapidly. Brain Research Bulletin, 1984, vol. 12, no. 1, pp. 143–146. https://doi.org/10.1016/0361-9230(84)90227-2
- Burleva E.P., Ektova M.V., Belentsov S.M., Chukin S.A., Makarov S.E., Veselov B.A. Treatment of lower extremity telangiectasias by thermocoagulation method using TS-3000 apparatus. Ambulatory Surgery (Russia), 2018, no. 1-2, pp. 72–79. (in Russian). https://doi.org/10.21518/1995-14772018-1-2-72-79
- Trelles M.A., Weiss R., Moreno-Moragas J., Romero C., Vélez M., Álvarez X. Treatment of leg veins with combined pulsed dye and Nd:YAG lasers: 60 patients assessed at 6 months. Lasers in Surgery and Medicine, 2010, vol. 42, no. 9, pp. 769–774. https://doi.org/10.1002/lsm.20972
- Dremin V., Zherebtsov E., Bykov A., Popov A., Doronin A., Meglinski I. Influence of blood pulsation on diagnostic volume in pulse oximetry and photoplethysmography measurements. Applied Optics, 2019, vol. 58, no. 34, pp. 9398–9405. https://doi.org/10.1364/ao.58.009398
- Bashkatov A.N., Genina E.A., Tuchin V.V., Altshuler G.B., Yaroslavsky I.V. Monte Carlo study of skin optical clearing to enhance light penetration in the tissue: implications for photodynamic therapy of acne vulgaris. Proceedings of SPIE, 2008, vol. 7022, pp. 80–91. https://doi.org/10.1117/12.803909
- Meglinski I.V., Matcher S.J. Quantitative assessment of skin layers absorption and skin reflectance spectra simulation in the visible and near-infrared spectral regions. Physiological Measurement, 2002, vol. 23, no. 4, pp. 741–753. https://doi.org/10.1088/0967-3334/23/4/312
- Kim O., McMurdy J., Lines C., Duffy S., Crawford G., Alber M. Reflectance spectrometry of normal and bruised human skins: experiments and modeling. Physiological Measurement, 2012, vol. 33, no. 2, pp. 159–175. https://doi.org/10.1088/0967-3334/33/2/159
- Meglinski I.V., Matcher S.J. Computer simulation of the skin reflectance spectra. Computer Methods and Programs in Biomedicine, 2003, vol. 70, no. 2, pp. 179–186. https://doi.org/10.1016/s0169-2607(02)00099-8
- Walters K.A., Roberts M.S. The structure and function of skin. Dermatological and Transdermal Formulations. CRC press, 2002, pp. 19–58.
- Shirshin E.A., Gurfinkel Y.I., Priezzhev A.V., Fadeev V.V., Lademann J., Darvin M.E. Two-photon autofluorescence lifetime imaging of human skin papillary dermis in vivo: assessment of blood capillaries and structural proteins localization. Scientific Reports, 2017, vol. 7, no. 1, pp. 1171. https://doi.org/10.1038/s41598-017-01238-w
- Wang S., Yu X.-Y., Fan W., Li C.-X., Fei W.-M., Li S., Zhou J., Hu R., Lui M., Xu F., Xu J., Cui Y. Detection of skin thickness and density in healthy Chinese people by using high‐frequency ultrasound. Skin Research and Technology, 2023, vol. 29, no. 1, pp. e13219. https://doi.org/10.1111/srt.13219
- Bashkatov A.N., Genina E.A., Tuchin V.V. Optical properties of skin, subcutaneous, and muscle tissues: a review. Journal of Innovative Optical Health Sciences, 2011, vol. 4, no. 1, pp. 9–38. https://doi.org/10.1142/S1793545811001319
- Ding H., Lu J.Q., Wooden W.A., Kragel P.J., Hu X.-H. Refractive indices of human skin tissues at eight wavelengths and estimated dispersion relations between 300 and 1600 nm. Physics in Medicine & Biology, 2006, vol. 51, no. 6, pp. 1479–1489. https://doi.org/10.1088/0031-9155/51/6/008
- Müller G.J., Roggan A. Laser-Induced Interstitial Thermotherapy. SPIE Press, 1995, 549 p.
- Wokalek H., Vanscheidt W., Martay K., Leder O. Morphology and localization of sunburst varicosities: an electron microscopic and morphometric study. The Journal of Dermatologic Surgery and Oncology, 1989, vol. 15, no. 2, pp. 149–154. https://doi.org/10.1111/j.1524-4725.1989.tb03021.x
- Lee S.H., Jeong S.K., Ahn S.K. An update of the defensive barrier function of skin. Yonsei Medical Journal, 2006, vol. 47, no. 3, pp. 293–306. https://doi.org/10.3349/ymj.2006.47.3.293
- Groen D., Gooris G.S., Barlow D.J., Lawrence M.J., Van Mechelen J.B., Demé B., Bouwstra J.A. Disposition of ceramide in model lipid membranes determined by neutron diffraction. Biophysical Journal, 2011, vol. 100, no. 6, pp. 1481–1489. https://doi.org/10.1016/j.bpj.2011.02.001
- Seteikin A.Yu. Model for calculating the temperature fields that appear when laser radiation acts on multilayer biological tissue. Journal of Optical Technology, 2005, vol. 72, no. 7, pp. 535–539. https://doi.org/10.1364/JOT.72.000535
- Labib R.S., Anhalt G.J., Patel H.P., Diaz L.A. Epidermal proteins. I. Differential extraction and quantitative polyacrylamide gel-electrophoretic analysis of basal spinous-cell proteins of neonatal mouse epidermis. Archives of Dermatological Research, 1985, vol. 277, no. 4, pp. 253–263. https://doi.org/10.1007/BF00509077
- Chaudhary P., Kumar A., Saxena N., Biswal U.C. Hyperbilirubinemia as a predictor of gangrenous/perforated appendicitis: a prospective study. Annals of Gastroenterology: Quarterly Publication of the Hellenic Society of Gastroenterology, 2013, vol. 26, no. 4, pp. 325–331.
- Malskies C.R., Eibenberger E., Angelopoulou E. The Recognition of ethnic groups based on histological skin properties. Proc. of the Vision, Modeling, and Visualization Workshop. Berlin, Germany, 2011, pp. 353–360. https://doi.org/10.2312/PE/VMV/VMV11/353-360
- Nicolaides N. Skin lipids. II. Lipid class composition of samples from various species and anatomical sites. Journal of the American Oil Chemists' Society, 1965, vol. 42, no. 8, pp. 691–702. https://doi.org/10.1007/BF02540042
- McMaster J.D., Jenkinson D.M., Noble R.C., Elder H.Y. The lipid composition of bovine sebum and dermis. British Veterinary Journal, 1985, vol. 141, no. 1, pp. 34–41. https://doi.org/10.1016/0007-1935(85)90124-1
- Waller J.M., Maibach H.I. Age and skin structure and function, a quantitative approach (II): protein, glycosaminoglycan, water, and lipid content and structure. Skin Research and Technology, 2006, vol. 12, no. 3, pp. 145–154. https://doi.org/10.1111/j.0909-752X.2006.00146.x
- Yannas I.V., Burke J.F., Gordon P.L., Huang C., Rubenstein R.H. Design of an artificial skin. II. Control of chemical composition. Journal of Biomedical Materials Research, 1980, vol. 14, no. 2, pp. 107–132. https://doi.org/10.1002/jbm.820140203
- Bonnett R., Davies J.E., Hursthouse M.B. Structure of bilirubin. Nature, 1976, vol. 262, no. 5566, pp. 326–328. https://doi.org/10.1038/262326a0
- Ermakov I.V., Gellermann W. Dermal carotenoid measurements via pressure mediated reflection spectroscopy. Journal of Biophotonics, 2012, vol. 5, no. 7, pp. 559–570. https://doi.org/10.1002/jbio.201100122
- Haynes W.M. CRC Handbook of Chemistry and Physics. CRC press, 2016, 2670 p.
- Thomas L.W. The chemical composition of adipose tissue of man and mice. Quarterly Journal of Experimental Physiology and Cognate Medical Sciences, 1962, vol. 47, no. 2, pp. 179–188. https://doi.org/10.1113/expphysiol.1962.sp001589
- Mujkić R., Mujkić D.Š., Ilić I., Rođak E., Šumanovac A., Grgić A., Divković D., Selthofer-Relatić K. Early childhood fat tissue changes—adipocyte morphometry, collagen deposition, and expression of CD163+ cells in subcutaneous and visceral adipose tissue of male children. International Journal of Environmental Research and Public Health, 2021, vol. 18, no. 7, pp. 3627. https://doi.org/10.3390/ijerph18073627
- Arenander E., Grimby G., Hallberg L., Westling H., Carlsten A. The volume and distribution of blood in patients with varicose veins. Scandinavian Journal of Clinical and Laboratory Investigation, 1962, vol. 14, no. 2, p. 170–178. https://doi.org/10.3109/00365516209079690
- Hale G.M., Querry M.R. Optical constants of water in the 200-nm to 200-μm wavelength region. Applied Optics, 1973, vol. 12, no. 3, pp. 555–563. https://doi.org/10.1364/AO.12.000555
- Calabro K. Modeling biological tissues in light tools Available at: https://www.synopsys.com/content/dam/synopsys/optical-solutions/documents/modeling-tissues-lighttools-paper.pdf (accessed: 09.05.2023)
- Lister T., Wright P.A., Chappell P.H. Optical properties of human skin. Journal of Biomedical Optics, 2012, vol. 17, no. 9, pp. 090901. https://doi.org/10.1117/1.JBO.17.9.090901
- Tozar T., Andrei I.R., Costin R., Pirvulescu R., Pascu M.L. Case series about ex vivo identification of squamous cell carcinomas by laser-induced autofluorescence and Fourier transform infrared spectroscopy. Lasers in Medical Science, 2018, vol. 33, no. 4, pp. 861–869. https://doi.org/10.1007/s10103-018-2445-5
- Sekar S.K.V., Bargigia I., Mora A.D., Taroni P., Ruggeri A., Tosi A., Farina A. Diffuse optical characterization of collagen absorption from 500 to 1700 nm. Journal of Biomedical Optics, 2017, vol. 22, no. 1, pp. 015006. https://doi.org/10.1117/1.JBO.22.1.015006
- Du H., Fuh R.-C.A., Li J., Corkan L.A., Lindsey J.S. PhotochemCAD: a computer‐aided design and research tool in photochemistry. Photochemistry and Photobiology, 1998. vol. 68, no. 2, pp. 141–142. https://doi.org/10.1111/j.1751-1097.1998.tb02480.x
- Bosschaart N., Edelman G.J., Aalders M.C.G., Van Leeuwen T.G., Faber D.J. A literature review and novel theoretical approach on the optical properties of whole blood. Lasers in Medical Science, 2014, vol. 29, no. 2, pp. 453–479. https://doi.org/10.1007/s10103-013-1446-7
- Sommer A., Van Mierlo P.L.H., Neumann H.A.M., Kessels A.G.H. Red and blue telangiectasias: Differences in oxygenation? Dermatologic Surgery, 1997, vol. 23, no. 1, pp. 55–59. https://doi.org/10.1111/j.1524-4725.1997.tb00009.x
- Khatun F., Aizu Y., Nishidate I. In vivo transcutaneous monitoring of hemoglobin derivatives using a red-green-blue camera-based spectral imaging technique. International Journal of Molecular Sciences, 2021, vol. 22, no. 4, pp. 1528. https://doi.org/10.3390/ijms22041528
- Kuenstner J.T., Norris K.H. Spectrophotometry of human hemoglobin in the near infrared region from 1000 to 2500 nm. Journal of Near Infrared Spectroscopy, 1994, vol. 2, no. 2, pp. 59–65. https://doi.org/10.1255/jnirs.32
- Al’tshuler G.B., Smirnov M.Z., Pushkareva A.E. Modeling of the laser and lamp treatment of telangiectasia. Optics and Spectroscopy, 2004, vol. 97, no. 1, pp. 141–144. https://doi.org/10.1134/1.1781295