Menu
Publications
2024
2023
2022
2021
2020
2019
2018
2017
2016
2015
2014
2013
2012
2011
2010
2009
2008
2007
2006
2005
2004
2003
2002
2001
Editor-in-Chief
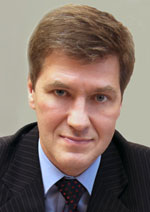
Nikiforov
Vladimir O.
D.Sc., Prof.
Partners
doi: 10.17586/2226-1494-2023-23-6-1106-1113
Dual-wavelength digital holographic interferometry for technical applications
Read the full article

Article in Russian
For citation:
Abstract
For citation:
Alekseenko I.V., Kozhevnikova A.M. Dual-wavelength digital holographic interferometry for technical applications. Scientific and Technical Journal of Information Technologies, Mechanics and Optics, 2023, vol. 23, no. 6, pp. 1106–1113 (in Russian). doi: 10.17586/2226-1494-2023-23-6-1106-1113
Abstract
A method of dual-wavelength digital holographic interferometry for analyzing and controlling surface shape for technical applications, including surfaces exposed to high-temperature plasma in fusion reactors, is presented. The capability of applying the method both using miniature vertical-emitting diodes (VCSEL) and conventional wavelength-tunable lasers is shown. The research method is based on dual-wavelength digital holographic interferometry, in which the phases of wave fronts reflected from the object detected at different wavelengths are compared to provide information about the shape of the object. Moreover, the sensitivity of the method is determined by the value of synthetic wavelength, which depends on the difference of wavelengths used for acquisition of digital holograms. The method used following wavelengths 854.000–854.082 nm and 779.900–779.870 nm. Implementation of vertical-emitting diodes for dual-wave holographic interferometry methods is shown. It is found that such diodes have a coherence length of about 20 cm and similar to He-Ne laser. The dependence of the emission wavelength of such sources on the current has been examined and it is determined that the output wavelength deviates less than 1 % during 24 hours. The application of the holographic method has been demonstrated for measuring the shape of objects used in various technical applications (a car body part and a shielding element of the internal wall of the Tokamak fusion reactor). The results of the research illustrate the opportunity to apply the technique of dual-wavelength holographic interferometry to measure a shape of the technical objects surface of various types. It should be noted that other method such as fringe projection can also be used to solve such problems, but it does not work sufficiently on low-scattering surfaces, in our case the lacquered surface of the car body, or specular reflecting surfaces. In addition, the LIDAR technique requires scanning the surface over time, which can lead to measurement errors if the object is unstable due to mechanical movements or vibrations. In dual- wavelength holographic interferometry such drawbacks can be reduced by short camera exposure times of milliseconds/ microseconds, or by using pulse laser with pulse durations of 10 ns. However, the major disadvantage of the method is the dependence of the mutual correlation of speckle structures of holograms on the wavelength difference. In order to increase the sensitivity of the method, it is necessary to increase this wavelength difference, and this can significantly reduce the signal-to-noise ratio and decrease the accuracy of the obtained data.
Keywords: digital holographic interferometry, digital holography, optical non-destructive testing
Acknowledgements. This article is performed in accordance with the support of the Russian Science Foundation (RSF), Project No. 23-79-00023 “Оptical Nondestructive Testing and Diagnostics of the First Wall of Tokamak Reactors Using Dual-Wavelength Digital Holographic Interferometry and Lidar” for the years 2023–2026.
References
Acknowledgements. This article is performed in accordance with the support of the Russian Science Foundation (RSF), Project No. 23-79-00023 “Оptical Nondestructive Testing and Diagnostics of the First Wall of Tokamak Reactors Using Dual-Wavelength Digital Holographic Interferometry and Lidar” for the years 2023–2026.
References
- Pedrini G., Zou Y.L., Tiziani H.J. Digital double-pulsed holographic interferometry for vibration analysis. Journal of Modern Optics, 1995, vol. 42, no. 2, pp. 367–374. https://doi.org/10.1080/09500349514550321
- Pedrini G., Zou Y.-L., Tiziani H.J. Simultaneous quantitative evaluation of in-plane and out-of-plane deformations by use of a multidirectional spatial carrier. Applied Optics, 1997, vol. 36, no. 4, pp. 786–792. https://doi.org/10.1364/ao.36.000786
- Pedrini G., Tiziani H., Zou Y. Digital holographic interferometry. Digital Speckle Pattern Interferometry and Related Techniques. Ed. by P.K. Rastogi. Chichester, N.Y., Wiley, 2001, pp. 337–362.
- Alexeenko I., Gusev M., Gurevich V. Separate recording of rationally related vibration frequencies using digital stroboscopic holographic interferometry. Applied Optics, 2009, vol. 48, no. 18, pp. 3475–3480. https://doi.org/10.1364/ao.48.003475
- Vandenrijt J.-F., Thizy C., Martin L., Beaumont F., Garcia J., Fabron C., Prieto É., Maciaszek T., Georges M.P. Digital holographic interferometry in the long-wave infrared and temporal phase unwrapping for measuring large deformations and rigid body motions of segmented space detector in cryogenic test. Optical Engineering, 2016, vol. 55, no. 12, pp. 121723–121723. https://doi.org/10.1117/1.oe.55.12.121723
- Dyomin V., Gribenyukov A., Davydova A., Zinoviev M., Olshukov A., Podzyvalov S., Polovtsev I., Yudin N. Holography of particles for diagnostics tasks. Applied Optics, 2019, vol. 58, no. 34, pp. G300–G310. https://doi.org/10.1364/ao.58.00g300
- Belashov A.V., Zhikhoreva A.A., Semenova I.V. Recording of long low-amplitude bulk elastic waves in transparent solid waveguides by digital and classical holography.Applied Sciences, 2022, vol. 12, no. 3, pp. 1687. https://doi.org/10.3390/app12031687
- Zou Y.L., Pedrini G., Tiziani H.J. Two-wavelength contouring with a pulsed ruby laser by employing TV-holography. Journal of Modern Optics, 1996, vol. 43, no. 3, pp. 653–646. https://doi.org/10.1080/09500349608232771
- Wagner C., Osten W., Seebacher S. Direct shape measurement by digital wavefront reconstruction and multi-wavelength contouring. Optical Engineering, 2000, vol. 39, no. 1, pp. 79–85. https://doi.org/10.1117/1.602338
- Carl D., Fratz M., Pfeifer M., Giel D.M., Höfler H. Multiwavelength digital holography with autocalibration of phase shifts and artificial wavelengths. Applied Optics, 2009, vol. 48, no. 34, pp. H1–H8. https://doi.org/10.1364/ao.48.0000h1
- Pitts R.A., Bonnin X., Escourbiac F., Frerichs H., Gunn J.P., Hirai T., Kukushkin A.S., Kaveeva E., Miller M.A., Moulton D., Rozhansky V., Senichenkov I., Sytova E., Schmitz O., Stangeby P.C., De Temmerman G., Veselova I., Wiesen S. Physics basis for the first ITER tungsten divertor. Nuclear Materials and Energy, 2019, vol. 20, pp. 100696. https://doi.org/10.1016/j.nme.2019.100696
- Razdobarin A.G., Gasparyan Y.M., Bogachev D.L., Dmitriev A.M., Elets D.I., Koval A.N., Kurskiev G.S., Mukhin E.E., Bulgadaryan D.G., Krat S.A., Marenkov E.D., Alekseenko I.V. Diagnostics complex of the first wall and divertor of tokamak with reactor technologies: control of erosion and temperature and monitoring of fusion fuel build-up. Plasma Physics Reports, 2022, vol. 48, no. 12, pp. 1389–1403. https://doi.org/10.1134/s1063780x22700283
- Schnars U., Juptner W. Digital Holography. Springer-Verlag and Heidelberg GmbH & Company K, 2005, 164 p.
- Takeda M., Ina H., Kobayashi S. Fourier-transform method of fringe-pattern analysis for computer-based topography and interferometry. Journal of the Optical Society of America, 1982, vol. 72. no. 1. pp. 156–160. https://doi.org/10.1364/josa.72.000156
- Friesem A.A., Levy U. Fringe formation in two-wavelength contour holography. Applied Optics, 1976, vol. 15, no. 12. pp. 3009–3020. https://doi.org/10.1364/ao.15.003009
- Kreis T. Handbook of Holographic Interferometry: Optical and Digital Methods. Wiley, 2005, 554 p.
- Claus D., Alekseenko I., Grabherr M., Pedrini G., Hibst R. Snap-shot topography measurement via dual-VCSEL and dual wavelength digital holographic interferometry. Light: Advanced Manufacturing, 2021. vol. 2, no. 4, pp. 403–414. https://doi.org/10.37188/lam.2021.029
- Pedrini G., Alekseenko I., Jagannathan G., Kempenaars M., Vayakis G., Osten W. Feasibility study of digital holography for erosion measurements under extreme environmental conditions inside the International Thermonuclear Experimental Reactor tokamak. Applied Optics, 2019, vol. 58, no. 5, pp. A147–A155. https://doi.org/10.1364/ao.58.00a147
- Goodman J.W. Wavelength and angle diversity. Speckle Phenomena in Optics: Theory and Applications. Greewood Village, Roberts and Company Publishers, 2006, pp. 153–169.