Menu
Publications
2024
2023
2022
2021
2020
2019
2018
2017
2016
2015
2014
2013
2012
2011
2010
2009
2008
2007
2006
2005
2004
2003
2002
2001
Editor-in-Chief
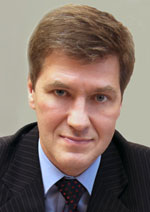
Nikiforov
Vladimir O.
D.Sc., Prof.
Partners
doi: 10.17586/2226-1494-2023-23-6-1223-1232
Convective heat transfer and hydrodynamics of flow at the endwall around a turbine blade under the influence of a magnetic field
Read the full article

Article in English
For citation:
Abstract
For citation:
Arjun K.S., Tide P.S. Convective heat transfer and hydrodynamics of flow at the endwall around a turbine blade under the influence of a magnetic field. Scientific and Technical Journal of Information Technologies, Mechanics and Optics, 2023, vol. 23, no. 6, pp. 1223–1232. doi: 10.17586/2226-1494-2023-23-6-1223-1232
Abstract
The present study analyses the influence of magnetohydrodynamics on endwall heat transfer in turbine blades using computational fluid dynamics simulations. The simulations consider the three-dimensional geometry of the turbine blade, the magnetic intensity, and the boundary conditions. The outcome revealed the existence of a magnetic field can outstandingly increase the pitch-averaged film cooling effectiveness and endwall heat transfer, particularly near the edges of the turbine vane with an optimal magnetic field. This results in a more uniform distribution of heat transfer along the endwall and can help to reduce hot spots and prevent thermal damage to the blade. The research also highlights the importance of considering the magnetic intensity and its impact on the flow characteristics and heat transfer when designing turbine blades for high-speed applications. By optimizing the design of the turbine blades to take into account the magnetohydrodynamic effect, engineers can improve the overall performance and lifespan of these critical components. Numerical simulations had been utilized to forecast the impacts of contouring of endwalls efficiently, employing the secondary kinetic energy coefficient as the accomplished parameter demonstrated in the current investigation. A reduction in endwall heat load with enhanced net heat flux reduction and aerodynamic performance is reported for a non-axisymmetrically contoured endwall subjected to optimal magnetic field strength. The novelty of the present study is the establishment of the impact of vortices on endwall heat transfer with respect to the vane under the influence of magnetohydrodynamics to reduce the weight and cost of a turbine engine.
Keywords: non-axisymmetric contouring, magnetohydrodynamics, net heat flux reduction, aerodynamic performance, secondary kinetic energy
References
References
- Arjun K.S., Tide P.S., Biju N. Optimum profiles of endwall contouring for enhanced net heat flux reduction and aerodynamic performance.Journal of Harbin Institute of Technology (New Series), 2023, in press. https://doi.org/10.11916/j.issn.1005-9113.2023037
- Krishna M.V., Ahamad N.A., Chamkha A.J. Numerical investigation on unsteady MHD convective rotating flow past an infinite vertical moving porous surface. Ain Shams Engineering Journal, 2021, vol. 12, no. 2, pp. 2099–2109. https://doi.org/10.1016/j.asej.2020.10.013
- Xenos M., Dimas S., Kafoussias N. MHD compressible turbulent boundary-layer flow with adverse pressure gradient. Acta Mechanica, 2005, vol. 177, pp. 171–190. https://doi.org/10.1007/s00707-005-0221-7
- Jaafar A., Waini I., Jamaludin A., Nazar R., Pop I. MHD flow and heat transfer of a hybrid nanofluid past a nonlinear surface stretching/shrinking with effects of thermal radiation and suction. Chinese Journal of Physics, 2022, vol. 79, pp. 13–27. https://doi.org/10.1016/j.cjph.2022.06.026
- Ali A., Kanwal T., Awais M., Shah Z., Kumam P., Thounthong P. Impact of thermal radiation and non-uniform heat flux on MHD hybrid nanofluid along a stretching cylinder. Scientific Reports, 2021, vol. 11, pp. 20262. https://doi.org/10.1038/s41598-021-99800-0
- Mobadersani F., Rezavand A. MHD effect on nanofluid flow and heat transfer in backward-facing step using two-phase model. AUT Journal of Mechanical Engineering, 2020, vol. 4, no. 1, pp. 51–66. https://doi.org/10.22060/AJME.2019.14843.5747
- Loganayagi V., Kameswaran P.K. Magnetohydrodynamic and heat transfer impacts on ferrofluid over a rotating disk: An application to hard disk drives. Journal of Thermal Science and Engineering Applications, 2021, vol. 13, no. 1, pp. 011001. https://doi.org/10.1115/1.4047007
- Nourbakhsh A., Mombeni H., Bayareh M. Effects of radiation and magnetohydrodynamics on heat transfer of nanofluid flow over a plate. SN Applied Sciences, 2019, vol. 1, pp. 1581. https://doi.org/10.1007/s42452-019-1634-6
- Ellahi R., Alamri S.Z., Basit A., Majeed A. Effects of MHD and slip on heat transfer boundary layer flow over a moving plate based on specific entropy generation. Journal of Taibah University for Science, 2018, vol. 12, no. 4, pp. 476–482. https://doi.org/10.1080/16583655.2018.1483795
- Ullah H., Hayat T., Ahmad S., Alhodaly M.S., Momani S. Numerical simulation of MHD hybrid nanofluid flow by a stretchable surface. Chinese Journal of Physics, 2021, vol. 71, pp. 597–609. https://doi.org/10.1016/j.cjph.2021.03.017
- Jusoh R., Nazar R., Pop I. Magnetohydrodynamic boundary layer flow and heat transfer of nanofluids past a bidirectional exponential permeable stretching/shrinking sheet with viscous dissipation effect. ASME Journal of Heat and Mass Transfer, 2019, vol. 141, no. 1, pp. 012406. https://doi.org/10.1115/1.4041800
- Yıldız F., Alpman E., Kavurmacioglu L., Camci C. An Artificial Neural Network (ANN) based aerothermal optimization of film cooling hole locations on the squealer tip of an HP turbine blade: preprint. SSRN Electronic Journal, 2022. https://doi.org/10.2139/ssrn.4117325
- Alam T., Kim M-H. A comprehensive review on single phase heat transfer enhancement techniques in heat exchanger applications. Renewable and Sustainable Energy Reviews, 2018, vol. 81, pp. 813–839. https://doi.org/10.1016/j.rser.2017.08.060
- Malvandi A. Film boiling of magnetic nanofluids (MNFs) over a vertical plate in presence of a uniform variable-directional magnetic field. Journal of Magnetism and Magnetic Materials, 2016, vol. 406, pp. 95–102. https://doi.org/10.1016/j.jmmm.2016.01.008
- Inverse Heat Conduction and Heat Exchangers.ed. by S. Bhattacharyya, R. Mehta, M.M. Ardekani, R. Biswas. London, UK, IntechOpen Ltd., 2020. http://dx.doi.org./10.5772/intechopen.80096
- Mahfoud B. Simulation of magnetic field effect on heat transfer enhancement of swirling nanofluid. International Journal of Computational Materials Science and Engineering, 2022, vol. 11, no. 4, pp. 2250007. https://doi.org/10.1142/S2047684122500075
- Arjun K.S., Tide P.S., Biju N. Turbine passage secondary flow dynamics and endwall heat transfer under different inflow turbulence. Journal of Harbin Institute of Technology (New Series), 2023, in press. https://doi.org/10.11916/j.issn.1005-9113.2023042
- Tsujita H., Mizuki S., Yamamoto A. Numerical investigation of effects of incidence angle on aerodynamic performance of ultra-highly loaded turbine cascade. Proc. of the ASME Turbo Expo2006: Power for Land, Sea, and Air, Turbomachinery, Parts A and B. Vol. 6,2006, pp. 839–849.https://doi.org/10.1115/GT2006-90939
- Snedden G.C. The Application of Non-Axisymmetric Endwall Contouring in a 1½ Stage, Rotating Turbine: PhD thesis, Durham University, United Kingdom, 2011.
- VázquezR., Fidalgo V.J. The effect of Reynolds and Mach number on end-wall profiling performance. Proc. of the ASME Turbo Expo 2010: Power for Land, Sea, and Air, Turbomachinery, Parts A, B, and C. Vol. 7, 2010, pp. 1357–1368.https://doi.org/10.1115/GT2010-22765
- Du K., Jia Y., Song H., Chen L., Zhang Q., Cui T., Liu C. Effect of slot jet flow on non-axisymmetric endwall cooling performance of high-load turbines. Machines, 2023, vol. 11, no. 2, pp. 134. https://doi.org/10.3390/machines11020134
- Schäflein L., Janssen J., Brandies H., Jeschke P., Behre S. Influence of purge flow injection on the performance of an axial turbine with three-dimensional airfoils and non-axisymmetric endwall contouring. Journal of Turbomachinery, 2023, vol. 145, no. 6, pp. 061004. https://doi.org/10.1115/1.4056238
- Menter F.R. Two-equation eddy-viscosity turbulence models for engineering applications. AIAA – Journal,1994, vol. 32, no. 8, pp. 1598–1605. https://doi.org/10.2514/3.12149
- Menter F.R., Langtry R.B., Likki S.R., Suzen Y.B., Huang P.G., Völker S. A correlation-based transition model using local variables - Part I: Model formulation. Journal of Turbomachinery, 2006, vol. 128, no. 3, pp. 413–422. https://doi.org/10.1115/1.2184352
- Lynch S.P., Thole K.A. Heat transfer and film cooling on a contoured blade endwall with platform gap leakage. Journal of Turbomachinery, 2017, vol. 139, no. 5, pp. 051002. https://doi.org/10.1115/1.4035202
- Ingram G. Endwall Profiling for the Reduction of Secondary Flow in Turbines. PhD thesis. Durham University, United Kingdom, 2003.
- Bai B., Li Z., Li J., Mao S., Ng W.F. The effects of axisymmetric convergent contouring and blowing ratio on endwall film cooling and vane pressure side surface phantom cooling performance. Journal of Engineering for Gas Turbines and Power, 2022, vol. 144, no. 2, pp. 021020. https://doi.org/10.1115/1.4052500
- Versteeg H.K., Malalasekera W. An Introduction to Computational Fluid Dynamics. The Finite Volume Method. Pearson Prentice Hall, 1995, 503 p.
- Snedden G., Dunn D., Backstrom V.T.W., Ingram G. Observations on the selection of objective function for the optimisation of turbine endwalls using computational fluid dynamics. Proc. of the 7th South African Conference on Computational and Applied Mechanics (SACAM), 2010, pp. 574–588.