Menu
Publications
2025
2024
2023
2022
2021
2020
2019
2018
2017
2016
2015
2014
2013
2012
2011
2010
2009
2008
2007
2006
2005
2004
2003
2002
2001
Editor-in-Chief
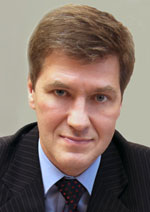
Nikiforov
Vladimir O.
D.Sc., Prof.
Partners
doi: 10.17586/2226-1494-2024-24-3-431-437
Lithium tetraborate co-doping with transition and alkali metals
Read the full article

Article in Russian
For citation:
Abstract
For citation:
Zakharchuk I.A., Daibagya D.S., Osadchenko A.V., Danilkin M.I., Ambrozevich S.A., Selyukov A.S. Lithium tetraborate co-doping with transition and alkali metals. Scientific and Technical Journal of Information Technologies, Mechanics and Optics, 2024, vol. 24, no. 3, pp. 431–437 (in Russian). doi: 10.17586/2226-1494-2024-24-3-431-437
Abstract
Luminescent properties of tissue-equivalent detectors have been studied. The detectors are made of lithium tetraborate and doped with magnesium, manganese, or tin. Analysis of the results obtained makes it possible to confirm the complexity of luminescence centers in lithium tetraborate without using structural analysis methods. For the first time, the effect of the method and order of introducing impurities on the storage properties of the studied materials was demonstrated and explained. The synthesis of the lithium tetraborate host occurred through the reaction of H3BO3 and Li2CO3. The binding agent was SiO2. Magnesium, manganese, or tin dopants were introduced during the synthesis of the host or later. The final stage of the synthesis was pressing of the powder into tablets and sintering in argon at 1158 K. The photoluminescence and pulsed cathodoluminescence signals were recorded by CCD-spectrometers. Portable pulsed electron accelerator was used for excitation of cathodoluminescence. Thermally stimulated luminescence was recorded by a special dosimetric reader with a heater and photo muliplier tube after exposure of the samples to an electron beam. Photoluminescence spectra, cathodoluminescence spectra, and glow curves were obtained for five samples of lithium tetraborate with various impurities, namely, magnesium, manganese, and tin. A comparison of the results was made to identify how the intensity signals depend on the amount of impurities and order of their introduction. It was found that the synthesis procedure and the order of introducing the dopants affect the luminescent properties of the materials. It has been suggested that the impurities take different positions in the lithium tetraborate structure. Predominant introduction of a particular impurity at a particular site leads to the following result. Doping with tin provides an increase in the number of luminescence centers of manganese and significantly sensitizes its luminescence, while doping with magnesium leads to the opposite result. The results indicate that it is possible to create advanced tissue-equivalent detectors with tailored luminescent. Depending on the dose of ionizing radiation, the use of material with different sensitivity and radiation resistance is required. Thus, the production of detectors based on lithium tetraborate and the described impurities in the future will give an opportunity to create a promising group of ionizing radiation detectors with various properties.
Keywords: photoluminescence, cathodoluminescence, thermally stimulated luminescence, lithium tetraborate, doping, tissueequivalence
References
References
- Sun S.K., Wang H.F., Yan X.P. Engineering persistent luminescence nanoparticles for biological applications: from biosensing/bioimaging to theranostics. Accounts of Chemical Research, 2018, vol. 51, no. 5, pp. 1131–1143. https://doi.org/10.1021/acs.accounts.7b00619
- McKeever S.W.S., Sholom S., Chandler J.R. Developments in the use of thermoluminescence and optically stimulated luminescence from mobile phones in emergency dosimetry. Radiation Protection Dosimetry, 2020, vol. 192, no. 2, pp. 205–235. https://doi.org/10.1093/rpd/ncaa208
- Christensen J.B., Togno M., Nesteruk K.P., Psoroulas S., Meer D., Weber D.Ch., Lomax T., Yukihara E.G., Safai S. Al2O3:C optically stimulated luminescence dosimeters (OSLDs) for ultra-high dose rate proton dosimetry. Physics in Medicine & Biology, 2021, vol. 66, no. 8, pp. 085003. https://doi.org/10.1088/1361-6560/abe554
- Stepanov V.A., Demenkov P.V., Nikulina O.V. Radiation hardening and optical properties of materials based on SiO2. Nuclear Energy and Technology, 2021, vol. 7. no. 2, pp. 145–155. https://doi.org/10.3897/nucet.7.69926
- Lin W., Boccard M., Zhong S., Paratte V., Jeangros Q., Antognini L., Dréon J., Cattin J., Thomet J., Liu Z., Chen Z., Liang Z., Gao P., Shen H., Ballif Ch. Degradation mechanism and stability improvement of dopant-free ZnO/LiFx/Al electron nanocontacts in silicon heterojunction solar cells. ACS Applied Nano Materials, 2020, vol. 3, no. 11, pp. 11391–11398. https://doi.org/10.1021/acsanm.0c02475
- Maye F., Turak A. LiF Nanoparticles Enhance Targeted Degradation of Organic Material under Low Dose X-ray Irradiation. Radiation, 2021, vol. 1, no. 2, pp. 131–144. https://doi.org/10.3390/radiation1020012
- Liu K., Velasquez B., Schüler E. High‐dose and ultra‐high dose rate (UHDR) evaluation of Al2O3:C optically stimulated luminescent dosimeter nanoDots and powdered LiF:Mg,Ti thermoluminescent dosimeters for radiation therapy applications. Medical Physics, 2024, vol. 51, no. 3, pp. 2311–2319. https://doi.org/10.1002/mp.16832
- Ravikumar N., Kumar R. A. Synthesis and thermoluminescence properties of Li2B4O7:Sm phosphor for dosimetry applications. Materials Today: Proceedings, 2019, vol. 18, part. 4, pp. 1716–1723. https://doi.org/10.1016/j.matpr.2019.05.268
- de Oliveira L.S.S., de Souza L.F., Donald G.G., D’Emidio M.F.S., De Lima Ferreira Novais A., Souza D. Challenges in personal and clinical dosimetry using Li2B4O7 and MgB4O7 as TLD and OSLD. Brazilian Journal of Radiation Sciences, 2022, vol. 10, no. 2A. https://doi.org/10.15392/bjrs.v10i2A.2019
- Chopra V., Dhoble S.J., Gupta K.K., Singh A., Pandey A. Thermoluminescence of Li2B4O7:Cu phosphor exposed to proton beam for dosimetric application. Radiation Measurements, 2018, vol. 118, pp. 108–115. https://doi.org/10.1016/j.radmeas.2018.05.002
- Danilkin M.I., Koksharov Yu.A., Romet I., Seeman V.O., Vereschagina N.Yu., Zubov A.I., Selyukov A.S. Manganese agglomeration and radiation damage in doped Li2B4O7. Radiation Measurements, 2019, vol. 126, pp. 106134. https://doi.org/10.1016/j.radmeas.2019.106134
- Shpotyuk O., Adamiv V., Teslyuk I., Ingram A., Demchenko P. Probing vacancy-type free-volume defects in Li2B4O7 single crystal by positron annihilation lifetime spectroscopy. Journal of Physics and Chemistry of Solids, 2018, vol. 112, pp. 8–13. https://doi.org/10.1016/j.jpcs.2017.08.025
- Mendoza-Anaya D., González-Romero A., Ávila O., González P.R., Escobar-Alarcón L. Thermally stimulated luminescence of Li2B4O7:Cu,Ag,P+PTFE. Journal of Luminescence, 2018, vol. 204, pp. 176–181. https://doi.org/10.1016/j.jlumin.2018.08.025
- Rahimi M., Zahedifar M., Sadeghi E. Synthesis, optical properties and thermoluminescence dosimetry features of manganese doped Li2B4O7 nanoparticles. Radiation Protection Dosimetry, 2018, vol. 181, no. 4, pp. 360–367. https://doi.org/10.1093/rpd/ncy035
- Ding C.C., Duan B. Investigations on the role of doped 3d transition ions in Li2B4O7 glasses: Local structures, spectroscopic properties and optical basicities. Materials Research Bulletin, 2022, vol. 156, pp. 111995. https://doi.org/10.1016/j.materresbull.2022.111995
- Kerikmäe M., Danilkin M., Lust A., Nagirnyi V., Pung L., Ratas A., Romet I., Seeman V. Hole traps and thermoluminescence in Li2B4O7:Be. Radiation Measurements, 2013, vol. 56, pp. 147–149. https://doi.org/10.1016/j.radmeas.2013.02.002
- Danilkin M.I., Vereschagina N.Yu., Vainer Yu.G., Kochiev M.V., Ambrozevich S.A., Romet I., Mändar H., Morozov A.N., Repeev Yu.A., Spassky D.A., Shutov A.V., Seleznev L.V., Mokrousova D.V., Selyukov A.S. Ultrafast and slow Mn2+ luminescence in lithium tetraborate. Journal of Alloys and Compounds, 2021, vol. 883, pp. 160852. https://doi.org/10.1016/j.jallcom.2021.160852
- Zakharchuk I.A., Danilkin M., Selyukov A., Ivkina O., Mosyagina I. Luminescent dosimetric materials based on magnesium tetraborate for proton beam metrology. ANRI (Apparatura i novosti radiacionnyh izmerenij), 2023, no. 3(114), pp. 45–55. (in Russian). https://doi.org/10.37414/2075-1338-2023-114-3-45-55
- Osadchenko A.V., Vashchenko A.A., Zakharchuk I.A., Daibagya D.S., Ambrozevich S.A., Volodin N.Yu., Cheptsov D.A., Dolotov S.M., Traven V.F., Avramenko A.I., Semenova S.L., Selyukov A.S. Organic light-emitting diodes with new dyes based on coumarin. Scientific and Technical Journal of Information Technologies, Mechanics and Optics, 2022, vol. 22, no. 6, pp. 1112–1118. (in Russian). https://doi.org/10.17586/2226-1494-2022-22-6-1112-1118
- Daibagya D.S., Ambrozevich S.A., Perepelitsa A.S., Zakharchuk I.A., Osadchenko A.V., Bezverkhnyaya D.M., Avramenko A.I., Selyukov A.S. Spectral and kinetic properties of silver sulfide quantum dots in an external electric field. Scientific and Technical Journal of Information Technologies, Mechanics and Optics, 2022, vol. 22, no. 6, pp. 1098–1103. (in Russian). https://doi.org/10.17586/2226-1494-2022-22-6-1098-1103
- van Bunningen A.J., Sontakke A.D., van der Vliet R., Spit V.G., Meijerink A. Luminescence temperature quenching in Mn2+ phosphors. Advanced Optical Materials, 2023, vol. 11, no. 6, pp. 2202794. https://doi.org/10.1002/adom.202202794
- Ye N. Structure design and crystal growth of UV nonlinear borate materials. Structure-Property Relationships in Non-Linear Optical Crystals I: The UV-Vis Region, 2012, pp. 181–221. https://doi.org/10.1007/430_2011_69
- Benavente J. F., Gómez-Ros J. M., Correcher V. Characterization of the thermoluminescence glow curve of Li2B4O7:Cu,Ag. Radiation Measurements, 2020, vol. 137, pp. 106427. https://doi.org/10.1016/j.radmeas.2020.106427
- Santos C., Lima A. F., Lalic M. V. First-principles study of structural, electronic, energetic and optical properties of substitutional Cu defect in Li2B4O7 scintillator. Journal of Alloys and Compounds, 2018, vol. 735, pp. 756–764. https://doi.org/10.1016/j.jallcom.2017.11.154