Menu
Publications
2025
2024
2023
2022
2021
2020
2019
2018
2017
2016
2015
2014
2013
2012
2011
2010
2009
2008
2007
2006
2005
2004
2003
2002
2001
Editor-in-Chief
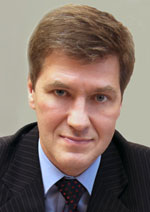
Nikiforov
Vladimir O.
D.Sc., Prof.
Partners
doi: 10.17586/2226-1494-2024-24-3-505-512
Restoration of unsteady heat flow from a thermal energy accumulator by solving the inverse heat conduction problem
Read the full article

Article in Russian
For citation:
Abstract
For citation:
Pilipenko N.V., Kolodiychuk P.A., Zakharova V.Yu., Faizullin R.O. Restoration of unsteady heat flow from a thermal energy accumulator by solving the inverse heat conduction problem. Scientific and Technical Journal of Information Technologies, Mechanics and Optics, 2024, vol. 24, no. 3, pp. 505–512 (in Russian). doi: 10.17586/2226-1494-2024-24-3-505-512
Abstract
This paper considers the problem restoring a non-stationary heat flow from a coolant to a heat-storing substance of a separate element of a thermal accumulator. Solving the problem allows avoiding errors associated with averaging the heat flow over all battery cells, and provides the opportunity to find the optimal sizes and composition of the filler for each battery cell. The problem is especially relevant for cascade batteries where cells with different fillers are simultaneously used. A comparison is made of two methods for solving the problem. The first method is based on numerical simulation of the thermal energy storage discharge process using the Computational Fluid Dynamics software package. The second approach proposed by the authors is based on the parametric identification of a differential-difference model of heat transfer with the solution of the inverse problem of heat conduction together with coefficient smoothing calculation. The proposed method makes it possible to smooth out abruptly changing thermophysical characteristics and take into account the moving phase boundary of a substance. The method for solving the inverse heat conduction problem can significantly reduce the recovery time of non-stationary boundary conditions of heat transfer for the entire battery and, thus, reduce the requirements for computing resources when designing and optimizing the battery by facilitating experimental search. For the first time, the use of the method of parametric identification and calculation of smoothing coefficients for solving the Stefan problem was considered and proposed. The results obtained can be used to calculate the heat flow from an individual element of a thermal energy accumulator.
Keywords: thermal energy accumulation, mathematical modeling, heat flow restoration, inverse heat conduction problem, Kalman filter
Acknowledgements. This study was supported by ITMO University as part of the project “Increasing the efficiency of energy systems through the use of thermal energy batteries” (No. 620150).
References
Acknowledgements. This study was supported by ITMO University as part of the project “Increasing the efficiency of energy systems through the use of thermal energy batteries” (No. 620150).
References
- Sorrell S. Reducing energy demand: A review of issues, challenges and approaches. Renewable and Sustainable Energy Reviews, 2015, no. 47, pp. 74–82. https://doi.org/10.1016/j.rser.2015.03.002
- Rahman A., Farrok O., Haque M.M. Environmental impact of renewable energy source based electrical power plants: Solar, wind, hydroelectric, biomass, geothermal, tidal, ocean, and osmotic. Renewable and Sustainable Energy Reviews, 2022, vol. 161, pp. 112279. https://doi.org/10.1016/j.rser.2022.112279
- Cirocco L., Pudney P., Riahi S., Liddle R., Semsarilar H., Hudson J., Bruno F. Thermal energy storage for industrial thermal loads and electricity demand side management. Energy Conversion and Management, 2022, vol. 270, pp. 116190. https://doi.org/10.1016/j.enconman.2022.116190
- Vakilaltojjar S.M., Saman W. Analysis and modelling of a phase change storage system for air conditioning applications. Applied Thermal Engineering, 2001, vol. 21, no. 3, pp. 249–263. https://doi.org/10.1016/S1359-4311(00)00037-5
- DNV GL. Energy Transition Outlook 2020 (A global and regional forecast to 2050). DNV GLAS Publications, 2020, pp. 44–46.
- Nada S., Alshaer W., Saleh R. Experimental investigation of PCM transient performance in free cooling of the fresh air of air conditioning systems. Journal of Building Engineering, 2020, vol. 29, pp. 101153. https://doi.org/10.1016/j.jobe.2019.101153
- Jin X., Wu F., Xu T., Huang G., Wu H., Zhou X., Wang D., Liu Y., Lai A.C. Experimental investigation of the novel melting point modified Phase–Change material for heat pump latent heat thermal energy storage application. Energy, 2021, vol. 216, pp. 119191. https://doi.org/10.1016/j.energy.2020.119191
- Kasibhatla R.R., Brüggemann D. Coupled conjugate heat transfer model for melting of PCM in cylindrical capsules. Applied Thermal Engineering, 2021, vol. 184, pp. 116301. https://doi.org/10.1016/j.applthermaleng.2020.116301
- Arkar C., Medved S. Influence of accuracy of thermal property data of a phase change material on the result of a numerical model of a packed bed latent heat storage with spheres. Thermochimica Acta, 2005, vol. 438, no. 1–2, pp. 192–201. https://doi.org/10.1016/j.tca.2005.08.032
- Cho K., Choi S.H. Thermal characteristics of paraffin in a spherical capsule during freezing and melting processes. International Journal of Heat and Mass Transfer, 2000, vol. 43, no. 17, pp. 3183–3196. https://doi.org/10.1016/S0017-9310(99)00329-4
- Benmansour A., Hamdan M.A., Bengeuddach A. Experimental and numerical investigation of solid particles thermal energy storage unit. Applied Thermal Engineering, 2006, vol. 26, no. 5–6, pp. 513–518. https://doi.org/10.1016/j.applthermaleng.2005.07.014
- Bédécarrats J.P., Strub F., Falcon B., Dumas J.P. Phase–change thermal energy storage using spherical capsules: performance of a test plant. International Journal of Refrigeration, 1996, vol. 19, no. 3, pp. 187–196. https://doi.org/10.1016/0140-7007(95)00080-1
- Zakharova V.Y., Faizullin R.O., Baranenko A.V., Kuznetsov P.A. Method for calculating latent heat thermal energy storage. Journal of International Academy of Refrigeration, 2021, no. 2(79), pp. 13–20. (in Russian). https://doi.org/10.17586/1606-4313-2021-20-2-13-20
- Mao Q., Cao W. Effect of variable capsule size on energy storage performances in a high-temperature three-layered packed bed system. Energy, 2023, vol. 273, pp. 127166. https://doi.org/10.1016/j.energy.2023.127166
- Gao L., Dong L., Liu Z., Gegentana, Che D., Sun B. Thermal performance analysis and multi-objective optimization of thermal energy storage unit with cascaded packed bed in a solar heating system. Applied Thermal Engineering, 2023, vol. 219, pp. 119416. https://doi.org/10.1016/j.applthermaleng.2022.119416
- Baturin O.V., Morozov I.I., Rabkesov I.V. Studying Hydrogasdynamic Flows Using the Universal Software Package Fluent. Samara, Samara University, 2007, 128 p. (in Russian)
- Pavlovskii V.A., Nikushchenko D.V. Computational hydrodynamics. Theoretical foundations. St. Petersburg, Lan' Publ., 2018, 368 p. (in Russian)
- Demenok S.L., Medvedev V.V., Sivukha S.M. Hydrodynamics and Heat Transfer in Spherical Packings. St. Petersburg, Strata Publ., 2012, 192 p. (in Russian)
- Kuznetcov P.A. Increasing the efficiency of air conditioning systems based on low-temperature energy storage. Dissertation for the degree of candidate of technical sciences. St. Petersburg, ITMO University, 2021. Available at: https://dissovet.itmo.ru/dissertation/?number=461692 (accessed: 17.02.2024). (in Russian)
- Zakharova V.Iu., Faizullin R.O., Baranenko A.V., Kalimzhanov D. Numerical and experimental studies of formic acid phase transitions as a heat-accumulating substance. Proc. of the Х International Scientific and Technical Conference “Low-Temperature and food Technologies in the 21st Century”, 2021, pp. 176–181. (in Russian)
- Patanka S. Numerical Heat Transfer and Fluid Flow. Washington, DC: CRC Press, 1980. 214 p. https://doi.org/10.1201/9781482234213
- Priyanshu G., Dutta A., Verma V., Thangamani I. Enthalpy porosity method for CFD simulation of natural convection phenomenon for phase change problems in the molten pool and its importance during melting of solids. Proc. of the COMSOL Conference in Bangalore, 2013.
- Pilipenko N.V. Uncertainty of non-stationary heat flux recovery by parametric identification of differential-difference model of heat transmission. Journal of Instrument Engineering, 2017, vol. 60, no. 7, pp. 664–671. (in Russian). https://doi.org/10.17586/0021-3454-2017-60-7-664-671
- Samarskii A.A., Moiseyenko B.D. An economic continuous calculation scheme for the stefan multidimensional problem. USSR Computational Mathematics and Mathematical Physics, 1965, vol. 5, no. 5, pp. 43–58. https://doi.org/10.1016/0041-5553(65)90004-2
- Pilipenko N.V., Zarichnyak Yu.P., Ivanov V.A., Khalyavin A.M. Parametric identification of differencial-difference models of heat transfer in one-dimensional bodies based on Kalman filter algorithms. Scientific and Technical Journal of Information Technologies, Mechanics and Optics, 2020, vol. 20, no. 4, pp. 584–588. (in Russian). https://doi.org/10.17586/2226-1494-2020-20-4-584-588
- Kirillov K., Pilipenko N. Solution algorithms for direct and backward heat conductivity problems by means of differential-difference models. Scientific and Technical Journal of Information Technologies, Mechanics and Optics, 2010, no. 5(69), pp. 106–109. (in Russian)
- Pilipenko N.V., Kolodiychuk P.A., Zarichnyak Yu.P. Differential-difference model of heat transfer in solids using the method of parametric identification. Scientific and Technical Journal of Information Technologies, Mechanics and Optics, 2022, vol. 22, no. 6, pp. 1237–1240. (in Russian). https://doi.org/10.17586/2226-1494-2022-22-6-1237-1240
- Kurbatova G.I. On the calculation of surfaces glaciation in seawater. Vestnik of Saint Petersburg University. Applied Mathematics. Computer Science. Control Processes, 2018, vol. 14, no. 3, pp. 186–199. (in Russian). https://doi.org/10.21638/11702/spbu10.2018.301
- Druzhinin P.V., Korichev A.A., Kosenkov I.A., Iurchik E.Iu. Mathematical model of the process of discharging a phase transition thermal accumulator. Tehniko-tehnologicheskie problemy servisa, 2009, no. 4(10), pp. 17–22. (in Russian)