Menu
Publications
2025
2024
2023
2022
2021
2020
2019
2018
2017
2016
2015
2014
2013
2012
2011
2010
2009
2008
2007
2006
2005
2004
2003
2002
2001
Editor-in-Chief
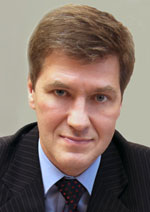
Nikiforov
Vladimir O.
D.Sc., Prof.
Partners
doi: 10.17586/2226-1494-2024-24-4-620-628
Computer simulation of heat and mass transfer processes during water vapor condensation from natural gas combustion products on smooth cylindrical tubes
Read the full article

Article in Russian
For citation:
Abstract
For citation:
Bryzgunov P.A., Rogalev A.N., Kindra V.O., Komarov I.I., Zlyvko O.V. Computer simulation of heat and mass transfer processes during water vapor condensation from natural gas combustion products on smooth cylindrical tubes. Scientific and Technical Journal of Information Technologies, Mechanics and Optics, 2024, vol. 24, no. 4, pp. 620–628 (in Russian). doi: 10.17586/2226-1494-2024-24-4-620-628
Abstract
The results of numerical simulation of heat and mass transfer processes during the condensation of water vapor from natural gas combustion products on bundles of smooth horizontal cylindrical tubes are presented. An empirical mathematical model of condensation of water vapor from a gas-steam mixture with a high content of non-condensable gases has been developed based on experimental data. The proposed mathematical model includes jointly solvable equations of thermal energy, momentum and mass conservation, while the equation of conservation of mass takes into account the species transport due to convection, molecular and turbulent diffusion. The phase change is taken into account in the source terms of the mass conservation equation; both condensation in the volume as the mixture passes through the dew point and local surface condensation on the cooling tubes are taken into account. To describe condensation in the volume, the return to saturation temperature model is used, and for surface condensation an algebraic empirical model was developed based on the analysis of experimental data. The advantage of the chosen approach is that there is no need to calculate the hydrodynamics of droplets and condensate films as a separate continuous one due to the influence of these factors on heat and mass transfer in the experimental coefficients, which significantly reduces the computational complexity of the problem and allows engineering calculations to be carried out in a coupled formulation. The structure of the developed mathematical model ensures easy integration with common commercial and freely available CFD codes. Based on experimental data, the coefficient of the developed condensation model was determined. It is shown that when adjusting the coefficient using one base point, the model ensures agreement with experimental data for other modes with a deviation not exceeding the experimental error. Using a verified model, a section of a condensation heat exchanger for gas turbine unit exhaust gases with a staggered bundle of smooth pipes in a coupled formulation was simulated, and the numerical value of increasing cooling water heat perception due to the utilization of latent heat of condensation was determined. The obtained modeling data and the developed model of condensation of water vapor from natural gas combustion products can be used in the calculations and design of condensing heat exchangers as well as condensing boilers.
Keywords: condensation of water vapor, non-condensable gases, drip condensation, condensation heat exchangers, exhaust heat recovery, heat and mass transfer processes, computational fluid dynamics
Acknowledgements. This study conducted by Moscow Power Engineering Institute was financially supported by the Ministry of Science and Higher Education of the Russian Federation (project No. FSWF-2023-0014, contract No. 075-03-2023-383, 2023/18/01).
References
Acknowledgements. This study conducted by Moscow Power Engineering Institute was financially supported by the Ministry of Science and Higher Education of the Russian Federation (project No. FSWF-2023-0014, contract No. 075-03-2023-383, 2023/18/01).
References
- Ionkin I.L., Roslyakov P.V., Luning B. Application of condensing heat utilizers at heat-power engineering objects (Review). Thermal Engineering, 2018, vol. 65, no. 10, pp. 677–690. https://doi.org/10.1134/s0040601518100038
- Apitsyna O.S., Malyshev A.A., Zaitsev A.V., Malinina O.S. Modeling of heat-hydrodynamic processes in evaporators of low-temperature systems with intrachannel boiling of refrigerants. Scientific and Technical Journal of Information Technologies, Mechanics and Optics, 2023, vol. 23, no. 2, pp. 422–429. (in Russian). https://doi.org/10.17586/2226-1494-2023-23-2-422-429
- Bonneau С., Josset С., Melot V., Auvity B. Comprehensive review of pure vapour condensation outside of horizontal smooth tubes. Nuclear Engineering and Design, 2019, vol. 349, pp. 92–108. https://doi.org/10.1016/j.nucengdes.2019.04.005
- Huang J., Zhang J., Wang L. Review of vapor condensation heat and mass transfer in the presence of non-condensable gas. Applied Thermal Engineering, 2015, vol. 89, pp. 469–484. https://doi.org/10.1016/j.applthermaleng.2015.06.040
- El Fil B., Kini G., Garimella S. A review of dropwise condensation: Theory, modeling, experiments, and applications. International Journal of Heat and Mass Transfer, 2020, vol. 160, pp. 120172. https://doi.org/10.1016/j.ijheatmasstransfer.2020.120172
- Tan Z., Cao Z., Chu W., Wang Q. Improvement on evaporation-condensation prediction of Lee model via a temperature deviation based dynamic correction on evaporation coefficient. Case Studies in Thermal Engineering, 2023, vol. 48, pp. 103147. https://doi.org/10.1016/j.csite.2023.103147
- Minko K.B., Artemov V.I., Klement'ev A.A. Simulation of condensation of stagnant or moving saturated vapor on a horizontal tube using the volume-of-fluid (VOF) method. Thermal Engineering, 2023, vol. 70, no. 3, pp. 175–193. https://doi.org/10.1134/s0040601523030059
- Yankov G.G., Milman O.O., Minko K.B., Artemov V.I. Simulation of the condensation processes of R113 in a horizontal pipe by the VOF method. Thermal Engineering, 2023, vol. 70, no. 11, pp. 860–874. https://doi.org/10.1134/s0040601523110137
- Minko K.B., Artemov V.I., Yan'kov G.G., Krylov V.S. Numerical simulation of steam condensation in a steam-gas mixture flow in a variable-section channel with a bundle of smooth horizontal tubes. Thermal Engineering, 2019, vol. 66, no. 12, pp. 928–935. https://doi.org/10.1134/s0040601519120061
- Minko K.B., Yankov G.G., Artemov V.I., Milman O.O. A mathematical model of forced convection condensation of steam on smooth horizontal tubes and tube bundles in the presence of noncondensables. International Journal of Heat and Mass Transfer, 2019, vol. 140, pp. 41–50. https://doi.org/10.1016/j.ijheatmasstransfer.2019.05.099
- Minko K.B., Artemov V.I., Yan'kov G.G., Krylov V.S. Verification of the mathematical model of steam film condensation from a flowing steam-air mixture on a bundle made of horizontal tubes. Thermal Engineering, 2019, vol. 66, no. 11, pp. 804–811. https://doi.org/10.1134/s004060151911003x
- Minko K.B., Yan'kov G.G., Krylov V.S., Klementiev A.A. An engineering model of steam condensation from a flowing steam-gas mixture on a bundle made of horizontal tubes. Thermal Engineering, 2021, vol. 68, no. 9, pp. 705–716. https://doi.org/10.1134/s0040601521080061
- Osakabe M., Itoh T., Yagi K. Condensation heat transfer of actual flue gas on horizontal tubes. Proceedings of the 5th ASME/JSME Joint Thermal Engineering Conference, San Diego, California, 1999, pp. 1–8.
- Lin С.-X., Wang D., Bao S. Numerical modeling and simulation of condensation heat transfer of a flue gas in a bundle of transport membrane tubes. International Journal of Heat and Mass Transfer, 2013, vol. 60, pp. 41–50. https://doi.org/10.1016/j.ijheatmasstransfer.2012.12.053
- Yang K., Yang J., Da Y., Han L., Deng L., Che D. A numerical study on convective condensation of flue gas in tubular heat exchangers. Applied Thermal Engineering, 2024, vol. 243, pp. 122524. https://doi.org/10.1016/j.applthermaleng.2024.122524
- Wu X., Che D. A numerical study of high moisture flue gas in tube banks. Numerical Heat Transfer, Part A: Applications, 2014, vol. 65, no. 4, pp. 357–377. https://doi.org/10.1080/10407782.2013.831673
- Vyskocil L., Schmid J., Macek J. CFD simulation of air–steam flow with condensation. Nuclear Engineering and Design, 2014, vol. 279, pp. 147–157. https://doi.org/10.1016/j.nucengdes.2014.02.014
- Menter F.R. Two-equation eddy-viscosity turbulence models for engineering applications. AIAA Journal, 1994, vol. 32, no. 8, pp. 1598–1605. https://doi.org/10.2514/3.12149
- Taniguchi H., Kudo K., Hwang Q.-R., Fujii A. Heat and mass transfer from air with high water vapor content (Latent heat recovery from flue gas). JSME international journal. Ser. 2, Fluids engineering, heat transfer, power, combustion, thermophysical properties, 1988, vol. 31, no. 2, pp. 299–305. https://doi.org/10.1299/jsmeb1988.31.2_299
- Osakabe M., Ishida K., Yagi K., Itoh T., Ohmasa K. Condensation heat transfer on tubes in actual flue gas. Heat Transfer – Asian Research, 2001, vol. 30, no. 2, pp. 139–151. https://doi.org/10.1002/1523-1496(200103)30:2<139::AID-HTJ5>3.0.CO;2-0