Меню
Публикации
2025
2024
2023
2022
2021
2020
2019
2018
2017
2016
2015
2014
2013
2012
2011
2010
2009
2008
2007
2006
2005
2004
2003
2002
2001
Главный редактор
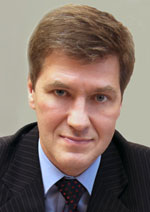
НИКИФОРОВ
Владимир Олегович
д.т.н., профессор
Партнеры
doi: 10.17586/2226-1494-2023-23-2-227-235
УДК 535.4
Анализ фазовых изображений, полученных при использовании голографической системы регистрации на основе эффекта геометрической фазы и поляризационной камеры
Читать статью полностью

Язык статьи - русский
Ссылка для цитирования:
Аннотация
Ссылка для цитирования:
Езерский А.С., Герасимов К.А., Мисюра А.А. Анализ фазовых изображений, полученных при использовании голографической системы регистрации на основе эффекта геометрической фазы и поляризационной камеры // Научно-технический вестник информационных технологий, механики и оптики. 2023. Т. 23, № 2. С. 227–235. doi: 10.17586/2226-1494-2023-23-2-227-235
Аннотация
Предмет исследования. Представлены результаты измерения глубины поверхности тестового объекта с применением цифровой голографии. Выполнено сравнение полученного изображения с моделью, построенной на основе документации к калибровочному слайду. Метод. В предложенном голографическом микроскопе вместо окуляра использована линза с эффектом геометрической фазы, которая преобразует пучок с линейной поляризацией в пару пучков с круговыми поляризациями (расходящийся и сходящийся). Для получения фазового распределения применен метод параллельного фазового сдвига. С помощью поляризационной камеры за одну экспозицию зарегистрировано четыре интерферограммы, соответствующие четырем линейным проекциям интерферирующих волн с правой и левой круговой поляризациями. Основные результаты. Получены голограммы фазового объект-микрометра, по которым методом параллельного фазового сдвига проведено восстановление распределения фазового запаздывания, вносимого объектом. Для коррекции аберрации применено вычитание зарегистрированного фазового набега освещающей волны — экспериментально полученной фазы волнового фронта без объекта. Практическая значимость. Разработанный цифровой голографический фазовый микроскоп на основе геометрической фазовой линзы и поляризационной камеры позволяет корректно визуализировать профиль рельефа поверхности. Микроскоп может найти применение в качестве инструмента для мониторинга состояния биологических объектов, подвергаемых внешнему воздействию.
Ключевые слова: цифровая голографическая микроскопия, голография, фазовое изображение, поляризационная камера, геометрическая фазовая линза, анализ фазовых распределений
Благодарности. Работа выполнена при поддержке гранта Президента Российской Федерации № MD-6101.2021.1.2.
Список литературы
Благодарности. Работа выполнена при поддержке гранта Президента Российской Федерации № MD-6101.2021.1.2.
Список литературы
-
ГОСТ Р 59321.3. Оптика и фотоника ГОЛОГРАФИЯ Часть 3. Голография цифровая и компьютерная. Термины и определения, 2021.
-
Kemper B., von Bally G. Digital holographic microscopy for live cell applications and technical inspection // Applied Optics. 2008. V. 47. N 4. P. A52. https://doi.org/10.1364/ao.47.000a52
-
Cacace T., Bianco V., Ferraro P. Quantitative phase imaging trends in biomedical applications // Optics and Lasers in Engineering. 2020. V. 135. P. 106188. https://doi.org/10.1016/j.optlaseng.2020.106188
-
Alam Z., Poddar R. An in-vivo depth-resolved imaging of developing zebrafish microstructure and microvasculature using swept-source optical coherence tomography angiography // Optics and Lasers in Engineering. 2022. V. 156. P. 107087. https://doi.org/10.1016/j.optlaseng.2022.107087
-
Kulya M.S., Balbekin N.S., Gredyuhina I.V., Uspenskaya M.V., Nechiporenko A.P., Petrov N.V. Computational terahertz imaging with dispersive objects // Journal of Modern Optics. 2017. V. 64. N 13. P. 1283–1288. https://doi.org/10.1080/09500340.2017.1285064
-
Kulya M., Semenova V., Gorodetsky A., Bespalov V.G., Petrov N.V. Spatio-temporal and spatiospectral metrology of terahertz broadband uniformly topologically charged vortex beams // Applied Optics. 2019. V. 58. N 5. P. A90. https://doi.org/10.1364/ao.58.000a90
-
Balbekin N.S., Kulya M.S., Belashov A.V., Gorodetsky A., Petrov N.V. Increasing the resolution of the reconstructed image in terahertz pulse time-domain holography // Scientific Reports. 2019. V. 9. N 1. P. 180. https://doi.org/10.1038/s41598-018-36642-3
-
Petrov N.V., Sokolenko B., Kulya M.S., Gorodetsky A., Chernykh A.V. Design of broadband terahertz vector and vortex beams: II. Holographic assessment // Light: Advanced Manufacturing. 2022. V. 3. N 44. https://doi.org/10.37188/lam.2022.044
-
Grachev Y.V., Kokliushkin V.A., Petrov N.V. Open-source 3D-printed terahertz pulse time-domain holographic detection module // Applied Optics. 2022. V. 61. N 5. P. B307. https://doi.org/10.1364/ao.444979
-
Khoroshun A.N., Chernykh A.V., Kucher S.V., Tsymbaluk A.N. Optimal parameters of a shearing interferometer with a singular light source // Journal of Optical Technology. 2012. V. 79. N 1. P. 9–11. https://doi.org/10.1364/jot.79.000009
-
Petrov N.V., Pavlov P.V., Malov A.N. Numerical simulation of optical vortex propagation and reflection by the methods of scalar diffraction theory // Quantum Electronics. 2013. V. 43. N 6. P. 582–587. https://doi.org/10.1070/qe2013v043n06abeh015190
-
Porfirev A.P., Khonina S.N. Simple method for efficient reconfigurable optical vortex beam splitting: erratum // Optics Express. 2017. V. 25. N 25. P. 32214. https://doi.org/10.1364/oe.25.032214
-
Khoroshun A.N., Chernyk A.V., Tsimbaluk A.N., Kirichenko J.A., Yezhov P.V., Kim J.-T. Experimental realization of an axial optical vortex beam synthesis using a Gaussian beam and two ramps from a spatial light modulator // Proceedings of SPIE. 2013. V. 9066. P. 90660P. https://doi.org/10.1117/12.2049059
-
Bekshaev A., Chernykh A., Khoroshun A., Masajada J., Popiołek-Masajada A., Riazantsev A. Controllable singular skeleton formation by means of the Kummer optical-vortex diffraction at a rectilinear phase step // Journal of Optics. 2021. V. 23. N 3. P. 034002. https://doi.org/10.1088/2040-8986/abcea7
-
Демин В.В., Макаров А.В., Половцев И.Г. Регистрация планктона с помощью имитатора погружаемой голографической камеры // Оптика атмосферы и океана. 2006. Т. 19. № 4. С. 312–318.
-
Бразовский В.В., Вагнер В.А., Евстигнеев В.В., Еськов А.В., Пролубников В.И., Тубалов Н.П. Голографический метод исследования дисперсного состава аэрозоля // Горизонты образования. 2006. № 8. С. 1–9.
-
Nikolaeva T.Y., Petrov N.V. Characterization of particles suspended in a volume of optical medium at high concentrations by coherent image processing // Optical Engineering. 2015. V. 54. N 8. P. 083101. https://doi.org/10.1117/1.oe.54.8.083101
-
Nikolaeva T.Y., Petrov N.V. Statistical study of coherent images of particles in the volume of optical medium // Proceedings of SPIE. 2014. V. 9216. P. 921612. https://doi.org/10.1117/12.2061671
-
Vovk T.A., Petrov N.V. Correlation characterization of particles in volume based on peak-to-basement ratio // Scientific Reports. 2017. V. 7. P. 43840. https://doi.org/10.1038/srep43840
-
Ларкин А.И. Визуализация и распознавание треков частиц методами когерентной лазерной голографии // Научная визуализация. 2018. Т. 10. № 1. С. 49–55. https://doi.org/10.26583/sv.10.1.03
-
Betin A.Y., Bobrinev V.I., Odinokov S.B., Evtikhiev N.N., Starikov R.S., Starikov S.N., Zlokazov E.Yu. Holographic memory optical system based on computer-generated Fourier holograms // Applied Optics. 2013. V. 52. N 33. P. 8142–8145. https://doi.org/10.1364/ao.52.008142
-
Hesselink L., Orlov S.S., Bashaw M.C. Holographic data storage systems // Proceedings of the IEEE. 2004. V. 92. N 8. P. 1231–1280. https://doi.org/10.1109/jproc.2004.831212
-
Cheremkhin P.A., Evtikhiev N.N., Krasnov V.V., Kulakov M.N., Kurbatova E.A., Molodtsov D.Y., Rodin V.G. Demonstration of digital hologram recording and 3D-scenes reconstruction in real-time // Proceedings of SPIE. 2016. V. 9889. P. 98891M. https://doi.org/10.1117/12.2227767
-
Ferraro P., Grilli S., Alfieri D., De Nicola S., Finizio A., Pierattini G., Javidi B., Coppola G., Striano V. Extended focused image in microscopy by digital holography // Optics Express. 2005. V. 13. N 18. P. 6738–6749. https://doi.org/10.1364/opex.13.006738
-
Nalegaev S.S., Belashov A.V., Petrov N.V. Application of photothermal digital interferometry for nonlinear refractive index measurements within a Kerr approximation // Optical Materials. 2017. V. 69. P. 437–443. https://doi.org/10.1016/j.optmat.2017.03.030
-
Momgaudis B., Guizard S., Bilde A., Melninkaitis A. Nonlinear refractive index measurements using time-resolved digital holography // Optics Letters. 2018. V. 43. N 2. P. 304–307. https://doi.org/10.1364/ol.43.000304
-
Petrov N.V., Nalegaev S.S., Belashov A.V., Shevkunov I.A., Putilin S.E., Lin Y.C., Cheng C.J. Time-resolved inline digital holography for the study of noncollinear degenerate phase modulation // Optics Letters. 2018. V. 43. N 15. P. 3481. https://doi.org/10.1364/ol.43.003481
-
Belashov A.V., Cheng C.-J., Petrov N.V. Noncollinear degenerate phase modulation in samples with inhomogeneous optical nonlinear properties [Invited] // Applied Optics. 2021. V. 60. N 10. P. B14–B2. https://doi.org/10.1364/AO.415102
-
Белашов А.В., Чжень Ч.-Ж., Петров Н.В. О возможности исследования доли неоднородностей оптических нелинейных сред с помощью осевой цифровой голографии с временным разрешением // Журнал технической физики. 2021. Т. 91. № 5. С. 846–854. https://doi.org/10.21883/JTF.2021.05.50699.340-20
-
Belashov A., Shevkunov I.A., Kolesova E.P., Orlova A.O., Putilin S.E., Veniaminov A.V., Cheng C.-J., Petrov N.V. Investigation of nonlinear optical properties of quantum dots deposited onto a sample glass using time-resolved inline digital holography // Journal of Imaging. 2022. V. 8. N 3. P. 74. https://doi.org/10.3390/jimaging8030074
-
Kumar V., Shakher C. Study of heat dissipation process from heat sink using lensless Fourier transform digital holographic interferometry // Applied Optics. 2015. V. 54. N 6. P. 1257–1266. https://doi.org/10.1364/AO.54.001257
-
Belashov A.V., Petrov N.V., Semenova I.V., Vasyutinskii O.S. Digital holographic micro-interferometry of nonradiative transitions in biological specimens // Proceedings of SPIE. 2015. V. 9529. P. 95290G. https://doi.org/10.1117/12.2184900
-
Creath K., Schwartz G.E. Dynamic visible interferometric measurement of thermal fields around living biological objects // Proceedings of SPIE. 2004. V. 5531. P. 24–31. https://doi.org/10.1117/12.562306
-
Конин Ю.А., Гаранин А.И., Перминов А.В. Дефектоскопия оптических волокон и заготовок методом голографической интерферометрии // Прикладная фотоника. 2015. Т. 2. № 2. С. 154–165.
-
Prisiazhniuk A.V., Sokolenko B.V., Poletaev D.A., Shostka N.V. Digital holographic testing of the optical fiber at welding area // Journal of Physics: Conference Series. 2019. V. 1400. N 6. P. 066042. https://doi.org/10.1088/1742-6596/1400/6/066042
-
Yamaguchi I., Kato J., Ohta S. Surface shape measurement by phase-shifting digital holography // Optical Review. 2001. V. 8. N 2. P. 85–89. https://doi.org/10.1007/s10043-001-0085-6
-
Yamaguchi I., Ohta S., Kato J. Surface contouring by phase-shifting digital holography // Optics and Lasers in Engineering. 2001. V. 36. N 5. P. 417–428. https://doi.org/10.1016/s0143-8166(01)00069-0
-
Dong J., Jia S., Jiang C. Surface shape measurement by multi-illumination lensless Fourier transform digital holographic interferometry // Optics Communications. 2017. V. 402. P. 91–96. https://doi.org/10.1016/j.optcom.2017.05.051
-
Belashov A.V., Petrov N.V. Improvement of rough surfaces height map reconstruction accuracy in tilt angle illumination digital holography // Optical Engineering. 2020. V. 59. N 10. P. 102414. https://doi.org/10.1117/1.oe.59.10.102414
-
Katkovnik V., Shevkunov I., Petrov N.V., Egiazarian K. Multiwavelength surface contouring from phase-coded noisy diffraction patterns: wavelength-division optical setup // Optical Engineering. 2018. V. 57. N 8. P. 085105. https://doi.org/10.1117/1.oe.57.8.085105
-
Verrier N., Alloul L., Gross M. Vibration of low amplitude imaged in amplitude and phase by sideband versus carrier correlation digital holography // Optics Letters. 2015. V. 40. N 3. P. 411–414. https://doi.org/10.1364/ol.40.000411
-
Тычинский В.П. Когерентная фазовая микроскопия внутриклеточных процессов // Успехи физических наук. 2001. Т. 171. № 6. С. 649–662. https://doi.org/10.3367/UFNr.0171.200106e.0649
-
Belashov A.V., Zhikhoreva A.A., Belyaeva T.N., Kornilova E.S., Petrov N.V., Salova A.V., Semenova I.V., Vasyutinskii O.S. Digital holographic microscopy in label-free analysis of cultured cells’ response to photodynamic treatment // Optics Letters. 2016. V. 41. N 21. P. 5035. https://doi.org/10.1364/ol.41.005035
-
Hu Y., Zuo C., Sun J., Chen Q., Zhang Y. A compact and lensless digital holographic microscope setup // Proceedings of SPIE. 2015. V. 9524. P. 952426. https://doi.org/10.1117/12.2189634
-
Popescu G. Quantitative Phase Imaging of Cells and Tissues. First edition. New York: McGraw-Hill Education, 2011. 384 p.
-
Montresor S., Picart P. Quantitative appraisal for noise reduction in digital holographic phase imaging // Optics Express. 2016. V. 24. N 13. P. 14322. https://doi.org/10.1364/oe.24.014322
-
Katkovnik V., Shevkunov I.A., Petrov N.V., Egiazarian K. Wavefront reconstruction in digital off-axis holography via sparse coding of amplitude and absolute phase // Optics Letters. 2015. V. 40. N 10. P. 2417–2420. https://doi.org/10.1364/ol.40.002417
-
Katkovnik V., Shevkunov I., Petrov N.V., Egiazarian K. High-accuracy off-axis wavefront reconstruction from noisy data: local least square with multiple adaptive windows // Optics Express. 2016. V. 24. N 22. P. 25068. https://doi.org/10.1364/oe.24.025068
-
Belashov A.V., Petrov N.V., Semenova I.V. Digital off-axis holographic interferometry with simulated wavefront // Optics Express. 2014. V. 22. N 23. P. 28363–28376. https://doi.org/10.1364/oe.22.028363
-
Bianco V., Memmolo P., Leo M., Montresor S., Distante C., Paturzo M., Picart P., Javidi B., Ferraro P. Strategies for reducing speckle noise in digital holography // Light: Science & Applications. 2018. V. 7. N 1. P. 1–16. https://doi.org/10.1038/s41377-018-0050-9
-
Choi K., Joo K.-I., Lee T.-H., Kim H.-R., Yim J., Do H., Min S.-W. Compact self-interference incoherent digital holographic camera system with real-time operation // Optics Express. 2019. V. 27. N 4. P. 4818. https://doi.org/10.1364/oe.27.004818
-
Rosen J., Vijayakumar A., Kumar M., Rai M.R., Kelner R., Kashter Y., Bulbul A., Mukherjee S. Recent advances in self-interference incoherent digital holography // Advances in Optics and Photonics. 2019. V. 11. N 1. P. 1–66. https://doi.org/10.1364/aop.11.000001
-
DeMars L.A., Mikuła-Zdańkowska M., Falaggis K., Porras-Aguilar R. Single-shot phase calibration of a spatial light modulator using geometric phase interferometry // Applied Optics. 2020. V. 59. N 13. P. D125–D130. https://doi.org/10.1364/ao.383610
-
Hong J., Kim M.K. Single-shot self-interference incoherent digital holography using off-axis configuration // Optics Letters. 2013. V. 38. N 23. P. 5196. https://doi.org/10.1364/ol.38.005196
-
Nguyen C.M., Muhammad D., Kwon H.-S. Spatially incoherent common-path off-axis color digital holography // Applied Optics. 2018. V. 57. N 6. P. 1504. https://doi.org/10.1364/ao.57.001504
-
Quan X., Matoba O., Awatsuji Y. Single-shot incoherent digital holography using a dual-focusing lens with diffraction gratings // Optics Letters. 2017. V. 42. N 3. P. 383. https://doi.org/10.1364/ol.42.000383
-
Nguyen T.H., Edwards C., Goddard L.L., Popescu G. Quantitative phase imaging with partially coherent illumination // Optics Letters. 2014. V. 39. N 19. P. 5511. https://doi.org/10.1364/ol.39.005511
-
Kumar M., Matoba O., Quan X., Rajput S.K., Awatsuji Y., Tamada Y. Single-shot common-path off-axis digital holography: applications in bioimaging and optical metrology [Invited] // Applied Optics. 2021. V. 60. N 4. P. A195. https://doi.org/10.1364/ao.404208
-
Bouchal P., Štrbková L., Dostál Z., Chmelík R., Bouchal Z. Geometric-phase microscopy for quantitative phase imaging of isotropic, birefringent and space-variant polarization samples // Scientific Reports. 2019. V. 9. N 1. P. 1–11. https://doi.org/10.1038/s41598-019-40441-9
-
Mico V., Zalevsky Z., García J. Common-path phase-shifting digital holographic microscopy: A way to quantitative phase imaging and superresolution // Optics Communications. 2008. V. 281. N 17. P. 4273–4281.
-
Hong J., Kim M. Overview of techniques applicable to self-interference incoherent digital holography // Journal of the European Optical Society: Rapid Publications. 2013. V. 8. P. 13077. https://doi.org/10.2971/jeos.2013.13077
-
Kim M.K. Incoherent digital holographic adaptive optics // Applied Optics. 2013. V. 52. N 1. P. A117–A130. https://doi.org/10.1364/ao.52.00a117
-
Shevkunov I., Petrov N.V. Phase retardation analysis in a rotated plane-parallel plate for phase-shifting digital holography // Journal of Imaging. 2022. V. 8. N 4. P. 87.
-
Awatsuji Y., Sasada M., Kubota T. Parallel quasi-phase-shifting digital holography // Applied Physics Letters. 2004. V. 85. N 6. P. 1069–1071.
-
Chernykh A.V., Ezerskii A.S., Georgieva A.O., Petrov N.V. Study on object wavefront sensing in parallel phase-shifting camera with geometric phase lens // Proceedings of SPIE. 2021. V. 11898. P. 118980X. https://doi.org/10.1117/12.2602841
-
Chernykh A.V., Georgieva A.O., Ezerskii A.S., Petrov N.V. Simple self-interference microscope design with geometric phase lens and polarization camera // Proc. of the Frontiers in Optics + Laser Science. 2021. P. JW7A.118.
-
Godden T.M., Muñiz-Piniella A., Claverley J.D., Yacoot A., Humphry M.J. Phase calibration target for quantitative phase imaging with ptychography // Optics Express. 2016. V. 24. N 7. P. 7679.
-
Shevkunov I., Georgieva A., Belashov A., Petrov N.V. Ptychography with DMD-based complex-valued probe // Proc. of the OSA Imaging and Applied Optics Congress (3D, COSI, DH, ISA, pcAOP). 2021. P. DM6C.6. https://doi.org/10.1364/DH.2021.DM6C.6
-
Choi K., Yim J., Min S.-W. Achromatic phase shifting self-interference incoherent digital holography using linear polarizer and geometric phase lens // Optics Express. 2018. V. 26. N 13. P. 16212–16225. https://doi.org/10.1364/oe.26.016212
-
Wolley O., Mekhail S., Moreau P.-A., Gregory T., Gibson G., Leuchs G., Padgett M.J. Imaging below the camera noise floor with a homodyne microscope // arXiv. 2022. arXiv:2208.04898. https://doi.org/10.48550/arXiv.2208.04898
-
Mikhail Polyanskiy. RefractiveIndex.INFO website [Электронныйресурс]. URL: https://refractiveindex.info/(дата обращения: 10.05.2008).
-
Georgieva A.O., Belashov A.V., Petrov N.V. Complex wavefront manipulation and holographic correction based on digital micromirror device: a study of spatial resolution and discretisation // Proceedings of SPIE. 2020. V. 11294. P. 112940B.
-
Georgieva A., Belashov A.V., Petrov N.V. Optimization of DMD-based independent amplitude and phase modulation by analysis of target complex wavefront // Scientific Reports. 2022. V. 12. N 1. P. 7754. https://doi.org/10.1038/s41598-022-11443-x
-
Georgieva A., Ezerskii A., Chernykh A., Petrov N. Numerical displacement of target wavefront formation plane with DMD-based modulation and geometric phase holographic registration system // Atmospheric and Oceanic Optics. 2022. V. 35. N 3. P. 258–265. https://doi.org/10.1134/s1024856022030034
-
Khonina S.N., Khorin P.A., Serafimovich P.G., Dzyuba A.P., Georgieva A.O., Petrov N.V. Analysis of the wavefront aberrations based on neural networks processing of the interferograms with a conical reference beam // Applied Physics B. 2022. V. 128. N 3. P. 60. https://doi.org/10.1007/s00340-022-07778-y
-
Khorin P.A., Serafimovich P.G., Dzyuba A.P., Georgieva A.O., Petrov N.V., Khonina S.N. Comparing of linear and conical interferograms for wavefront aberrations analysis based on neural networks // Proceedings of SPIE. 2022. V. 12295. P. 122950Q. https://doi.org/10.1117/12.2630978
-
Linarès-Loyez J., Ferreira J.S., Rossier O., Lounis B., Giannone G., Groc L., Cognet L., Bon P. Self-interference (SELFI) microscopy for live super-resolution imaging and single particle tracking in 3D // Frontiers in Physics. 2019. V. 7. P. 68. https://doi.org/10.3389/fphy.2019.00068
-
Егорова Д.А., Куликов А.В., Мухтубаев А.Б. Метод и технология доставки оптического излучения к биологическим микрообъектам // Научно-технический вестник информационных технологий, механики и оптики. 2017. Т. 17. № 5. С. 775–781. https://doi.org/10.17586/2226-1494-2017-17-5-775-781