Menu
Publications
2024
2023
2022
2021
2020
2019
2018
2017
2016
2015
2014
2013
2012
2011
2010
2009
2008
2007
2006
2005
2004
2003
2002
2001
Editor-in-Chief
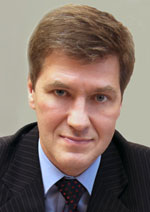
Nikiforov
Vladimir O.
D.Sc., Prof.
Partners
doi: 10.17586/2226-1494-2024-24-3-438-447
Analysis of chemical interactions during filling a cesium vapor cell for a quantum magnetometer
Read the full article

Article in Russian
For citation:
Abstract
For citation:
Yulmetova O.S., Shcherbak A.G., Reshetnyak P.E., Zavitaev A.S., Shevchenko A.N., Yulmetova R.F. Analysis of chemical interactions during filling a cesium vapor cell for a quantum magnetometer. Scientific and Technical Journal of Information Technologies, Mechanics and Optics, 2024, vol. 24, no. 3, pp. 438–447 (in Russian). doi: 10.17586/2226-1494-2024-24-3-438-447
Abstract
The results of the development and research of the technological process for manufacturing spherical vapor cells are presented. Such cells are used in quantum devices, such as magnetometers, gyroscopes, and atomic clocks. Their work is based on optical pumping and detection of the state of alkali metal vapors, in particular cesium. To increase the lifetime of cesium spin polarization in the vapor cell, it is filled with a buffer inert gas. The quality of cell manufacturing directly affects such device characteristics as the width of resonance lines and the achievable signal-to-noise ratio. The proposed cell manufacturing technology simplifies the technological process, eliminates the use of specialized equipment, and increases the reproducibility of results associated with chemical reactions of an alkali metal with foreign impurities in a buffer gas. This is achieved by detecting the formed compounds and excluding them from the composition of the gaseous environment of the cells through the selection of a reasonable sequence of technological operations in the cell manufacturing cycle. The use of traditional methods of X-ray diffraction is associated with the need to depressurize the vapor cell with cesium which leads to the inevitable reaction of cesium with components of the air environment. The work proposes a two-stage analytical assessment of the composition of the gas mixture. At the first stage, the thermodynamic resolution of all possible reactions in the cesium-nitrogen-impurity oxygen system is determined. At the second stage, the color spectrum of the spectra of experimentally obtained reaction products is compared with the color of the products of thermodynamically allowed interactions. Thermodynamic analysis based on a two-stage approach made it possible to identify the formation of cesium suboxides in a vapor cell when it was heated in the temperature range of 273–700 K. To exclude them from the composition of the vapor cell, a sequence of operations was proposed. It involves the formation of an ampoule using the glass blowing method which has a technological cylindrical part and a spherical cell connected to it by a constriction. High purity cesium encapsulated in a glass shell is placed into the technological part of the ampoule, after which the ampoule is evacuated. After opening the capsule with cesium, thermal distillation of pure cesium into a spherical zone takes place. The technological process is completed by filling the cell with buffer gas, after which it is sealed off. The absence of heating during filling a vapor cell with nitrogen significantly simplifies the technological process and minimizes the amount of foreign impurities in the form of cesium suboxides in the gas mixture.
Keywords: vapor cell, cesium, cesium suboxides, buffer gas, Gibbs energy, chemical thermodynamics, laser spectroscopy
Acknowledgements. Authors express their gratitude to Michel A.G. for manufacturing ampoules using the glass blowing method, Dementyev M.M. for assistance in vacuamizing and filling ampoules with nitrogen as well as carrying out spectral measurements.
References
Acknowledgements. Authors express their gratitude to Michel A.G. for manufacturing ampoules using the glass blowing method, Dementyev M.M. for assistance in vacuamizing and filling ampoules with nitrogen as well as carrying out spectral measurements.
References
- Vershovskii A.K., Litmanovich Yu.A., Pazgalev A.S., Peshekhonov V.G. Nuclear magnetic resonance gyro: ultimate parameters. Gyroscopy and Navigation, 2018, vol. 9, no. 3, pp. 162–176. https://doi.org/10.1134/S2075108718030100
- Pei H., Duan L., Ma L., Fan S., Cai Z., Wu Z., Fan W., Quan W. Real-time quantum control of spin-coupling damping and application in atomic spin gyroscopes. Cell Reports Physical Science, 2024, vol. 5, no. 2, pp. 101832. https://doi.org/10.1016/j.xcrp.2024.101832
- Li R., Quan W., Fan W., Xing L., Fang J. Influence of magnetic fields on the bias stability of atomic gyroscope operated in spin-exchange relaxation-free regime. Sensors and Actuators A: Physical, 2017, vol. 266, pp. 130–134. https://doi.org/10.1016/j.sna.2017.09.023
- Jie S., Liu Z., Wang J., Zhang S., Zhao K. Calibration of the coil constants and nonorthogonal angles of triaxial NMR coils based on in-situ EPR magnetometers. Journal of Magnetic Resonance, 2024, vol. 360, pp. 107634. https://doi.org/10.1016/j.jmr.2024.107634
- Ranjbaran M., Tehranchi M.M., Hamidi S.M., Khalkhali S.M.H. Relaxation time dependencies of optically detected magnetic resonance harmonics in highly sensitive Mx magnetometers. Journal of Magnetism and Magnetic Materials, 2019, vol. 469, pp. 522–530. https://doi.org/10.1016/j.jmmm.2018.09.031
- Knapkiewicz P. Technological assessment of MEMS alkali vapor cells for atomic references. Micromachines, 2019, vol. 10, no. 1, pp. 25. https://doi.org/10.3390/mi10010025
- Wolters J., Buser G., Horsley A., Béguin L., Jöckel A., Jahn J.P., Warburton R.J., Treutlein P. Simple atomic quantum memory suitable for semiconductor quantum dot single photons. Physical Review Letters, 2017, vol. 119, no. 6, pp. 060502. https://doi.org/10.1103/PhysRevLett.119.060502
- Katz O., Firstenberg O. Light storage for one second in room-temperature alkali vapor. Nature Communications, 2018, vol. 9, pp. 2074. https://doi.org/10.1038/s41467-018-04458-4
- Zugenmaier M., Dideriksen K.B., Sørensen A.S., Albrecht B., Polzik E.S. Long-lived non-classical correlations towards quantum communication at room temperature. Communications Physics, 2018, vol. 1, pp. 76. https://doi.org/10.1038/s42005-018-0080-x
- Kobtsev S., Radnatarov D., Khripunov S., Popkov I., Andryushkov V., Steshchenko T., Lunin V., Zarudnev Y. Feedback-controlled and digitally processed coherent population trapping resonance conversion in 87Rb vapour to high-contrast resonant peak. New Journal of Physics, 2017, vol. 19, no. 4, pp. 043016. https://doi.org/10.1088/1367-2630/aa68b9
- Chi H., Quan W., Zhang J., Zhao L., Fang J. Advances in anti-relaxation coatings of alkali-metal vapor cells. Applied Surface Science, 2020, vol. 501, pp. 143897. https://doi.org/10.1016/j.apsusc.2019.143897
- Wang X., Li J., Liu Z., Wu Z., Shao Y. Optimizing 129Xe and 131Xe relaxation in an NMR gyroscope using buffer gas pressure and wall coating. Journal of Magnetic Resonance, 2023, vol. 352, pp. 107430. https://doi.org/10.1016/j.jmr.2023.107430
- Shevchenko A.N., Zakharova E.A. Research of magnetic resonance quality factor dependence on cesium distribution in quantum rotation sensor cell. Scientific and Technical Journal of Information Technologies, Mechanics and Optics, 2019, vol. 19, no. 4, pp. 567–573. (in Russian). https://doi.org/10.17586/2226-1494-2019-19-4-567-573
- Woetzel S., Talkenberg F., Scholtes T., IJsselsteijn R., Schultze V., Meyer H.-G. Lifetime improvement of micro-fabricated alkali vapor cells by atomic layer deposited wall coatings. Surface & Coatings Technology, 2013, vol. 221, pp. 158–162. https://doi.org/10.1016/j.surfcoat.2013.01.044
- Gibbs J.W., Bumstead H.A., Van Name R.G., Longley W.R. The Collected Works of J. Willard Gibbs: In two volumes, Longmans, Green and Co., 1928.
- Scherbak A.G., Yulmetova O.S. Contrast image formation based on thermodynamic approach and surface laser oxidation process for optoelectronic read-out system. Optics and Laser Technology, 2018, vol. 101, pp. 242–247. https://doi.org/10.1016/j.optlastec.2017.11.030
- Tit M.A., Yulmetova O.S., Sisyukov A.N. Analysis of niobium thin film modification based on thermodynamic approach. Proc. of the 2020 IEEE Conference of Russian Young Researchers in Electrical and Electronic Engineering (EIConRus), 2020, pp. 1049–1052. https://doi.org/10.1109/EIConRus49466.2020.9038932
- Yulmetova O.S., Scherbak A.G. Composition analysis of thin films formed on beryllium surfaces under pulsed laser action by the method of chemical thermodynamics. The International Journal of Advanced Manufacturing Technology, 2018, vol. 97, no. 9-12, pp. 3231–3236. https://doi.org/10.1007/s00170-018-2216-2
- Tit M.A., Yulmetova O.S., Andreeva V.D., Sisyukov A.N., Yulmetova R.F. Application of niobium nitride thin films for improvement of performance characteristics of cryogenic gyroscope. Proc. of the 2021 IEEE Conference of Russian Young Researchers in Electrical and Electronic Engineering (ElConRus), 2021, pp. 1232–1235. https://doi.org/10.1109/ElConRus51938.2021.9396714
- Pliushchev V.E., Stepin B.D. Chemistry and technology of lithium, rubidium and cesium compounds. Moscow, Himija Publ., 1970, 408 p. (in Russian)
- Vlasova M.A., Chalkov V.V., Shevchenko A.N. Special aspects of the VCSEL lasers use in an installation for monitoring the parameters of quantum sensors gas cells. Proc. of the XI Congress of Young Scientists. Vol. 1, St. Petersburg, ITMO University, 2022, pp. 68–71. (in Russian)
- Lebedev V., Hartwing S., Middelmann T. Fast and robust optically pumped cesium magnetometer. Advanced Optical Technologies, 2020, vol. 9, no. 5, pp. 275–286. https://doi.org/10.1515/aot-2020-0024
- Bison G., Wynands R., Weis A. Optimization and performance of an optical cardiomagnetometer. Journal of the Optical Society of America B, 2005, vol. 22, no. 1, pp. 77–85. https://doi.org/10.1364/JOSAB.22.000077
- Fabricant A., Novikova I., Bison G. How to bild a magnetometer with thermal atomic vapor: a tutorial. New Journal of Physics, 2023, vol. 25, no. 2, pp. 025001. https://doi.org/10.1088/1367-2630/acb840
- Vershovskii A.K., Dmitriev S.P., Pazgalev A.S., Petrenko M.V., Kozlov G.G. Projection spin noise in optical quantum sensors based on thermal atoms. Technical Physics, 2020, vol. 65, no. 8. pp. 1193–1203. https://doi.org/10.1134/S1063784220080204
- Bagal L.I. Chemistry and technology of primary explosives. Moscow, Mashinostroenie Publ., 1975, 456 p. (in Russian)
- Heimel S. Thermodynamic Properties of Cesium up to 1500oK. NASA TN D-2906. 1965. 33 p.
- Lokshin E.P., Voskoboinikov N.B. Rubidium and cesium. Apatity, Kola Science Centre of the Russian Academy of Sciences, 1996, 168 p. (in Russian)
- Patton B., Ishikawa K., Jau Y.-Y., Happer W. Intrinsic impurities in glass alkali-vapor cells. Physical Review Letters, 2007, vol. 99, no. 2, pp. 027601. https://doi.org/10.1103/PhysRevLett.99.027601
- Sokolov A.N., Schedrinsky M.Z., Bebyakov V.M., Vorobev M.G., Sinkova V.A., Sukharev K.N., Rozanov L.N. Study of partial pressures of gases in vacuum chamber using multifunctional high-vacuum plant. Proceedings of Saint Petersburg Electrotechnical University, 2021, no. 3, pp. 5–10. (in Russian)
- Korovin N.V., Maslennikova G.N., Mingulina E.I., Filippov E.L. General chemistry course. Moscow, Vysshaja shkola, 1990, pp. 109–110, 140–141. (in Russian)
- Pitz G.A., Wertepny D.E., Perram G.P. Pressure broadening and shift of the cesium D1 transition by the noble gases and N2, H2, HD, D2, CH4, C2H6, CF4, and 3He. Physical Review A, 2009, vol. 80, no. 6, pp. 062718. https://doi.org/10.1103/PHYSREVA.80.062718