Menu
Publications
2024
2023
2022
2021
2020
2019
2018
2017
2016
2015
2014
2013
2012
2011
2010
2009
2008
2007
2006
2005
2004
2003
2002
2001
Editor-in-Chief
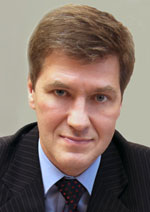
Nikiforov
Vladimir O.
D.Sc., Prof.
Partners
doi: 10.17586/2226-1494-2021-21-4-578-591
Solution of super- and hypersonic gas dynamic problems with a model of high-temperature air
Read the full article

Article in Russian
For citation:
Abstract
For citation:
Volkov K.N., Dobrov Yu.V., Karpenko A.G., Yakovchuk M.S. Solution of super- and hypersonic gas dynamic problems with a model of high-temperature air. Scientific and Technical Journal of Information Technologies, Mechanics and Optics, 2021, vol. 21, no. 4, pp. 578–591 (in Russian). doi: 10.17586/2226-1494-2021-21-4-578-591
Abstract
The study considers the solution of a number of problems of supersonic and hypersonic gas dynamics using a model that takes into account the dissociation and ionization of air. The results of verification and validation of the developed numerical method using various difference schemes (the Roe scheme, Rusanov scheme, AUSM scheme) for discretizing convective flows are presented. The formulation of the mathematical model for high-temperature air uses the presence of equilibrium chemical reactions of dissociation and ionization. For this purpose, at high incoming flow velocities, the Kraiko model is applied, which includes equilibrium chemical reactions in air at high temperatures. To discretize the basic equations, the finite volume method on an unstructured grid is applied. One of the features of the constructed mathematical model is the implementation of the transition between physical and conservative variables. Relationships are given, with the help of which the transition from conservative variables to physical ones and vice versa is carried out when using the high-temperature air model. To ensure the stability of numerical calculations, an entropy correction is introduced. The decrease in entropy in the solution of hyperbolic equations is excluded by introducing an artificial viscosity according to Neumann, as well as by using the Godunov method with an exact solution of the Riemann problem and methods based on the approximate solution of the problem of the decay of an arbitrary discontinuity. A number of problems of supersonic gas dynamics (supersonic flow in a channel with a straight step and supersonic flow around a sphere) are numerically solved taking into account high-temperature effects. The criteria for the accuracy of numerical calculations related to the location of shock-wave structures are discussed. The calculated shock-wave structure of the flow is compared with the data available in the literature, as well as with calculations using the perfect gas model. Some results of numerical calculations are compared with the available experimental data. The shock-wave flow patterns obtained in the framework of the inviscid model, which takes into account the effect of viscosity and its dependence on temperature, and the turbulent flow model are compared. On the basis of numerical simulation data, the influence of viscous effects on the flow characteristics in a channel with a straight step and hypersonic flow around a sphere is considered. The influence of various numerical factors on the shape of the bow shock and the presence of fluctuations in the solution behind the shock is emphasized. As part of the work, a computational module was prepared for the commercial package Ansys Fluent, implemented with the help of user programming tools. The prepared module expands the standard capabilities of commercial software focused on solving computational gas dynamics problems, and is available to Ansys Fluent users for solving hypersonic aerodynamics problems. The developed means of numerical simulation can be useful in the design and optimization of hypersonic aircraft.
Keywords: mathematical modeling, aerodynamics, supersonic flow, shock wave, real gas, channel
Acknowledgements. The study was financially supported by the Russian Science Foundation (project No. 19-71-10019).
References
Acknowledgements. The study was financially supported by the Russian Science Foundation (project No. 19-71-10019).
References
-
Volkov K.N., Deriugin Iu.N., Emelianov V.N., Kozelkov A.S., Karpenko A.G., Teterina I.V. Acceleration of Gasdynamic Calculations on Unstructured Grids.Moscow, Fizmatlit Publ., 2013, 536 p. (in Russian)
-
Lipanov A.M., Karskanov S.A. Application of high-order schemes for modeling the process of braking of supersonic flows in rectangular channels. Computational Continuum Mechanics, 2013, vol. 6, no. 3, pp. 292–299. (in Russian)
-
Zabarко D.А., Коtenev V.P. Numerical study of laminar flows of viscid chemically-reactive gas near blunted bodies. Herald of the Bauman Moscow State Technical University. Series Natural Sciences, 2006, no. 1, pp. 77–95. (in Russian)
-
Kraiko A.N., Makarov V.E. Explicit analytic formulas defining the equilibrium composition and thermodynamic functions of air for temperatures from 200 to 20000 K. High Temperature,1996, vol. 34, no. 2, pp. 202–213.
-
Volkov K.N., Dobrov Yu.V., Karpenko A.G., Malkovsky S.I., Sorokin A.A. Simulation of gas dynamics of hypersonic aircrafts with the use of model of high-temperature air and graphics processor units. Numerical Methods and Programming, 2021, vol. 22, no. 1, pp. 29–46. (in Russian). https://doi.org/10.26089/NumMet.v22r103
-
Kulikovskii A.G., Pogorelov N.V., Semenov A.Iu. Mathematical Aspects of Numerical Solution of Hyperbolic Systems. Moscow, Fizmatlit Publ., 2001, 607 p. (in Russian)
-
Roe P.L. Approximate Riemann solvers, parameter vectors, and difference schemes. Journal of Computational Physics, 1981, vol. 43, no. 2, pp. 357–372. https://doi.org/10.1016/0021-9991(81)90128-5
-
RusanovV.V. The calculation of the interaction of non-stationary shock waves and obstacles. USSR Computational Mathematics and Mathematical Physics, 1961, vol. 1, no. 2, pp. 304–320. https://doi.org/10.1016/0041-5553(62)90062-9
-
Liou M.-S. A sequel to AUSM. Part II. AUSM+-up for all speeds. Journal of Computational Physics, 2006, vol. 214, no. 1, pp. 137–170. https://doi.org/10.1016/j.jcp.2005.09.020
-
Bulat P.V., Volkov K.N. WENO schemes for solution of unsteady one-dimensional gas dynamics test problems. Scientific and Technical Journal of Information Technologies, Mechanics and Optics, 2016, vol. 16, no. 1, pp. 174–180. (in Russian). https://doi.org/10.17586/2226-1494-2016-16-1-174-180
-
Bulat M.P., Volobuev I.A., Volkov K.N., Pronin V.A. Numerical simulation of regular and mach reflection of shock wave from the wall. Scientific and Technical Journal of Information Technologies, Mechanics and Optics, 2017, vol. 17, no. 5, pp. 920–928. (in Russian). https://doi.org/10.17586/2226-1494-2017-17-5-920-928
-
Deryugin Yu.N., Zhuchkov R.N., Zelenskiy D.K., Kozelkov A.S., Sarazov A.V., Kudimov N.F., Lipnickiy Yu.M., Panasenko A.V., Safronov A.V. Validation results for the LOGOS multifunction software package in solving problems of aerodynamics and gas dynamics for the lift-off and injection of launch vehicles. Mathematical Models and Computer Simulations, 2015, vol. 7, no. 2, pp. 144–153. https://doi.org/10.1134/S2070048215020052
-
Kozelkov A.S., Kurulin V.V., Lashkin S.V., Shagaliev R.M., Yalozo A.V. Investigation of supercomputer capabilities for the scalable numerical simulation of computational fluid dynamics problems in industrial applications. Computational Mathematics and Mathematical Physics, 2016, vol. 56, no. 8, pp. 1506–1516. https://doi.org/10.1134/S0965542516080091
-
Woodward P.R., Colella P. The numerical simulation of two-dimensional fluid flow with strong shocks. Journal of Computational Physics, 1984, vol. 54, no. 1, pp. 115–173. https://doi.org/10.1016/0021-9991(84)90142-6
-
MacCormack R.W. Carbuncle computational fluid dynamics problem for blunt-body flows. Journal of Aerospace Information Systems, 2013, vol. 10, no. 5, pp. 229–239. https://doi.org/10.2514/1.53684
-
Isaev S.A., Lysenko D.A. Testing of the Fluent package in calculation of supersonic flow in a step channel. Journal of Engineering Physics and Thermophysics, 2004, vol. 77, no. 4, pp. 857–860. https://doi.org/10.1023/B:JOEP.0000045174.69463.6f
-
Isaev S.A., Lysenko D.A. Testing of numerical methods, convective schemes, algorithms for approximation of flows, and grid structures by the example of a supersonic flow in a step-shaped channel with the use of the CFX and Fluent packages. Journal of Engineering Physics and Thermophysics, 2009, vol. 82, no. 2, pp. 321–326. https://doi.org/10.1007/s10891-009-0187-8
-
Berthon C. Robustness of MUSCL schemes for 2D unstructured meshes. Journal of Computational Physics, 2006, vol. 218, no. 2, pp. 495–509. https://doi.org/10.1016/j.jcp.2006.02.028
-
Christov I., Popov B. New non-oscillatory central schemes on unstructured triangulations for hyperbolic systems of conservation laws. Journal of Computational Physics, 2008, vol. 227, no. 11, pp. 5736–5757. https://doi.org/10.1016/j.jcp.2008.02.007
-
Holt M., Hoffman G.H. Calculation of hypersonic flow past sphere and ellipsoids. American Rocket Society, 1961, No. 61-209-1903.
-
Lobb R.K. Experimental measurement of shock detachment distance on spheres fired in air at hypervelocities. AGARDograph, 1964, vol. 68, pp. 519–527. https://doi.org/10.1016/B978-1-4831-9828-6.50031-X
-
Zhluktov S.V., Smekhov G.D., Tirskii G.A. Rotation-vibration-dissociation interaction in a multicomponent nonequilibrium viscous shock layer. Fluid Dynamics, 1994, vol. 29, no. 6, pp. 876–887. https://doi.org/10.1007/BF02040799
-
Volkov K.N., Emelyanov V.N., Karpenko A.G. Numerical simulation of gas dynamic and physical-chemical processes in hypersonic flows past bodies. Numerical Methods and Programming, 2017, vol. 18, no. 4, pp. 387–405. (in Russian). https://doi.org/10.26089/NumMet.v18r433
-
Emelyanov V.N., Karpenko A.G., Volkov K.N. Simulation of hypersonic flows with equilibrium chemical reactions on graphics processor units. Acta Astronautica, 2019, vol. 163, part A, pp. 259–271. https://doi.org/10.1016/j.actaastro.2019.01.010
-
Golovachev Iu.P. Numerical Simulation of Viscous Gas Flows in Shock Layers. Moscow, Nauka Publ., 1996, 376 p. (in Russian)