Menu
Publications
2025
2024
2023
2022
2021
2020
2019
2018
2017
2016
2015
2014
2013
2012
2011
2010
2009
2008
2007
2006
2005
2004
2003
2002
2001
Editor-in-Chief
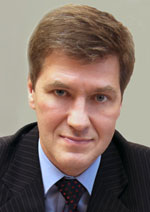
Nikiforov
Vladimir O.
D.Sc., Prof.
Partners
doi: 10.17586/2226-1494-2021-21-5-785-790
A balanced algorithm of the hybrid large-particle method and its verification on some test problems
Read the full article

Article in Russian
For citation:
Abstract
For citation:
Sadin D.V. A balanced algorithm of the hybrid large-particle method and its verification on some test problems. Scientific and Technical Journal of Information Technologies, Mechanics and Optics, 2021, vol. 21, no. 5, pp. 785–790 (in Russian). doi: 10.17586/2226-1494-2021-21-5-785-790
Abstract
In series of numerical experiments involving the Shu-Osher problem of nonlinear acoustics and the Woodward-Colella problem of two interacted blast waves, the author studied the computational properties of a new algorithm for the hybrid large-particle method. The numerical method is a two-step predictor-corrector type in time. Spatial derivatives are split by physical processes. At the first stage of splitting, the gradient and deformation terms of the conservation laws are taken into account, and at the second stage, convective flows are taken into account. The proposed balanced algorithm of the method includes a more dissipative upwind reconstruction of fluxes at the “predictor” step and a centered (non-dissipative on smooth solutions) approximation at the correction step: CDP2-UC (Customizable Dissipative Properties — Upwind-Centered). For a more flexible control of the numerical viscosity, a nonlinear correction of the scheme based on a parametric combination of known limiters is implemented. The numerical scheme has a second-order approximation in space and time on smooth solutions. The balanced algorithm of the hybrid large-particle method demonstrated a monotonic solution with a qualitative resolution of the details of the gas flow in the entire domain of determining the test problems. No spurious oscillations occurred during the process of fining the mesh, and convergence to the reference density profile was observed. The influence of a limiter on the numerical dissipation of the CDP2-UC scheme is analyzed. The results present the comparison with the following variants of the schemes: MUSCL (Monotone Upstream Scheme for Conservation Laws), MUSCL-CABARET with a NOLD limiter (Non-Oscillatory Low-Dissipative), the discontinuous Galerkin method with various forms of nonlinear correction, the hybrid weighted nonlinear scheme of the fourth order of approximation (CCSSR-HW4) and the popular WENO5 scheme (Weighted Essentially Non-Oscillatory Scheme) with fifth order of accuracy. The proposed algorithm successfully competes with modern numerical methods that have a formally higher (fourth and fifth) order of approximation. The hybrid large-particle method has the simplicity, uniformity, and cost-effectiveness of the algorithm, as well as high resolution. The test calculations allowed the author to estimate the range of parametric control of the numerical dissipation of the method for correct numerical modeling of the applied problems with nonlinear wave fields and strong shock waves.
Keywords: hybrid large-particle method, balanced algorithm, accuracy, convergence, Shu–Osher problem, Woodward–Colella problem
References
References
1. Godunov S.K. A finite difference method for the computation of discontinuous solutions of the equations of fluid dynamics. Mathematics of the USSR - Sbornik, 1959, vol. 47, no. 8-9, pp. 357–393.
2. Toro E.F. Riemann solvers and numerical methods for fluid dynamics: A Practical Introduction. Berlin, Heidelberg, Springer-Verlag, 2009, 724 p. https://doi.org/10.1007/b79761
3. Cockburn B., Shu C.-W. Runge–Kutta discontinuous Galerkin methods for convection-dominated problems. Journal of Scientific Computing, 2001, vol. 16, no. 3, pp. 173–261. https://doi.org/10.1023/A:1012873910884
4. Ladonkina M.E., Neklyudova O.A., Tishkin V.F. Application of the RKDG method for gas dynamics problems. Mathematical Models and Computer Simulations, 2014, vol. 6, no. 4, pp. 397–407. https://doi.org/10.1134/S207004821404005X.
5. Liu X.-D., Osher S., Chan T. Weighted essentially non-oscillatory schemes. Journal of Computational Physics, 1994, vol. 115, no. 1, pp. 200–212. https://doi.org/10.1006/jcph.1994.1187
6. Bulat P.V., Volkov K.N. WENO schemes for solution of unsteady one-dimensional gas dynamics test problems. Scientific and Technical Journal of Information Technologies, Mechanics and Optics, 2016, vol. 16, no. 1, pp. 174–180. (in Russian). https://doi.org/10.17586/2226-1494-2016-16-1-174-180
7. Fedorenko R.P. The application of difference schemes of high accuracy to the numerical solution of hyperbolic equations. USSR Computational Mathematics and Mathematical Physics, 1963, vol. 2, no. 6, pp. 1355–1365. https://doi.org/10.1016/0041-5553(63)90351-3
8. Liu X., Zhang S., Zhang H., Shu C.-W. A new class of central compact schemes with spectral-like resolution II: Hybrid weighted nonlinear schemes. Journal of Computational Physics, 2015, vol. 284, pp. 133–154. https://doi.org/10.1016/j.jcp.2014.12.027
9. Harten A. High resolution schemes for hyperbolic conservation laws. Journal of Computational Physics, 1983, vol. 49, no. 3, pp. 357–393. https://doi.org/10.1016/0021-9991(83)90136-5
10. Rodionov A.V. A comparison of the CABARET and MUSCL-type schemes. Mathematical Models and Computer Simulations, 2014, vol. 6, no. 2, pp. 203–225. https://doi.org/10.1134/S2070048214020094
11. Kurganov A., Liu Y. New adaptive artificial viscosity method for hyperbolic systems of conservation laws. Journal of Computational Physics, 2012, vol. 231, no. 24, pp. 8114–8132. https://doi.org/10.1016/j.jcp.2012.07.040
12. Popov I., Sukov S. Modified method of adaptive artificial viscosity for solution of gas dynamics problems on parallel computer systems. EPJ Web of Conferences, 2018, vol. 173, pp. 03020. https://doi.org/10.1051/epjconf/201817303020
13. Woodward P.R., Colella P. The numerical simulation of two-dimensional fluid flow with strong shocks. Journal of Computational Physics, 1984, vol. 54, no. 1, pp. 115–173. https://doi.org/10.1016/0021-9991(84)90142-6
14. Shu C.-W., Osher S. Efficient implementation of essentially non-oscillatory shock-capturing schemes, II. Journal of Computational Physics, 1989, vol. 83, no. 1, pp. 32–78. https://doi.org/10.1016/0021-9991(89)90222-2
15. Sadin D.V. TVD scheme for stiff problems of wave dynamics of heterogeneous media of nonhyperbolic nonconservative type. Computational Mathematics and Mathematical Physics, 2016, vol. 56, no. 12, pp. 2068–2078. https://doi.org/10.1134/S0965542516120137
16. Sadin D.V. Schemes with customizable dissipative properties as applied to gas-suspensions flow simulation. Matematicheskoe modelirovanie, 2017, vol. 29, no. 12, pp. 89–104. (in Russian)
17. Sadin D.V. Application of scheme with customizable dissipative properties for gas flow calculation with interface instability evolution. Scientific and Technical Journal of Information Technologies, Mechanics and Optics, 2018, vol. 18, no. 1, pp. 153–157. (in Russian). https://doi.org/10.17586/2226-1494-2018-18-1-153-157
18. Sadin D.V. A modification of the large-particle method to a scheme having the second order of accuracy in space and time for shockwave flows in a gas suspension. Bulletin of the South Ural State University, Series: Mathematical Modelling, Programming and Computer Software, 2019, vol. 12, no. 2, pp. 112–122. (in Russian). https://doi.org/10.14529/mmp190209
19. Sadin D.V., Davidchuk V.A. Comparison of a modified large-particle method with some high resolution schemes. one-dimensional test problems. Numerical Methods and Programming, 2019, vol. 20, no. 2, pp. 138–146. (in Russian). https://doi.org/10.26089/NumMet.v20r214
20. Sadin D.V. Analysis of dissipative properties of a hybrid large-particle method for structurally complicated gas flows. Computer Research and Modeling, 2020, vol. 12, no. 4, pp. 757–772. (in Russian). https://doi.org/10.20537/2076-7633-2020-12-4-757-772